Flash and JavaScript are required for this feature.
Download the track from iTunes U or the Internet Archive.
Description: This lecture is the third of three on the neocortex and covers the fiber architecture, thalamocortical organization and connections of neocortex.
Instructor: Gerard E. Schneider
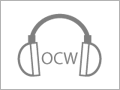
Lec 37: Neocortex, part 3
The following content is provided under a Creative Commons license. Your support will help MIT OpenCourseWare continue to offer high quality educational resources for free. To make a donation or view additional materials from hundreds of MIT courses, visit MIT OpenCourseWare at ocw.mit.edu.
PROFESSOR: Yeah, that's an exciting time in graduate school.
[LAUGHTER]
When you're finished with classes.
AUDIENCE: I am 26 years old. I'm done with taking classes. It's all research from here.
PROFESSOR: Look, I don't want to wait for others to show up because we-- as it is, we're probably short one class in going through all the neocortex very carefully. I just want to point out major things that I think you need to know.
When we talk about radial vesicles in the neocortex we just mean these. You see on the previous picture here? These are the slides from last time. We started last time here. These are the radial vesicles. These are transverse groups of axons.
So what kind of axon has to be in there? That's what I'm asking you. And if you've learned a bit about neuronanatomy you shouldn't have to even look at the book. They've got to be thalamocorticals. They're terminating in all the layers, but especially in layer 4 when you see the layers numbered out here.
All right. And what else do they include? Well, various outputs. There are outputs to the brain stem that are coming from layer 5. There are outputs to the thalamus coming from layer 6. And there's outputs to other areas of cortex coming from layer 2 and 3.
So that's what makes up those radial vesicles. In fact, there's also some intracolumnar axons. But most of those are probably not in the radial vesicles but axons that have so little myelin that you don't really see them in this myelin stain.
So I just summarized that in the following slides here. And I mentioned the line of Gennari. It's a sort of historical point, but it's an interesting one because it refers to one of these-- this one. This transverse group of axons we can see here on the picture from Rodale and there's a similar one in the book.
So just mention what that is there. If you look at the-- you can actually see it in a dissection if you make a slice through the visual cortex. You see this light area. And I'll mark it here. This is in a young human brain. It's actually a little clearer.
The purpose of these slides was to show the distinct nature of the cytoarchitecture of these different regions. And the clearest boundary in all the cortex is the boundary between area 17 and 18. 17 on the left here, 18 on the right.
This is in a myelin stain. Well, you see, again, big difference in the left side, which is area 17, and the right side, which is area 18. And in the middle of 17 you have all these axons. So they have to be mostly transverse axons. Some short association axons and perhaps some longer ones that go from one part of area 17 to others.
And this is from the adult. And here I've numbered in the Nissl stain the layers for you. You see that here-- this is Gennari's line here, the axons in the middle of layer 4. So there's a lot of granule cells on either side of it. And there you see-- these are corresponding pictures. This is the myelin where you see those-- that stripe of Gennari, named after the medical student who first noticed it, called attention to it.
So now let's talk about the specialized cortical columns. We used to think they were mainly in places like visual cortex where the two eyes are represented in adjacent stripes or columns of cortex.
So how was that visualized? Do you remember? The injected a tracer right into the eyeball. It was taken up by the retinal ganglion cells. And then they use a tracer like proline that will go [INAUDIBLE] So it reaches the geniculate body. It gets taken up by the cells in the geniculate, which then it's transferred up to the-- transported up to the visual cortex.
And if you do a tangential sections of the cortex and put together different sections, you can get a picture like this that shows that zebra stripe pattern. The light stripes here are the labeled ones from one eye. And if you had labeled both eyes, it would be totally white. But there the other eye projects to the in between stripes there.
But it turns out that columnar types of termination of axons is very common in the cortex. Nauta, and this is-- he worked with Pat Goldman. She came up here to his lab and learned some of the techniques for tracing. And this is from a study they did together where they were tracing prefrontal association area projections to more posterior parts of the cortex. And they traced them here to the retrosplenial area of the cortex. And you'll see this termination in stripes.
And I just point out here at the top, I wrote this morning-- columnar patterns of axon termination are a common feature of many inputs to neocortical areas. Many of the transcortical projections are like this, where you have more than one transcortical input coming in to the same area. And some of them are also-- the callosal projections also terminate in this matter, indicating some kind of integration of these two different inputs.
Now we've talked a number of different times about major types of cortex. First of all, the major types are neocortex and the other cortex and the in between ones. Paralimbic areas are often-- sometimes people call them neocortex. Most people just call them juxtalo cortex. Transitional areas between neocortex and limbic system structures.
So those are the three major types. But within those types now, there are different regions. We know about Broca's areas. They are all different areas in the neocortex and he did it by cytoarchitecture with Nissl stains.
You can also use major connections as for grouping them. So let's look at examples of that. If you just use connections, as Mesulam was doing when he grouped the paralimbic areas, you see one group-- I probably showed this before-- one group that projects to parahippocampal area. So it's sending information towards the hippocampus.
And the other group-- and together they constitute the entire limbic lobe-- the other area is darker in his picture here. They use different colors in the book, but I used a-- I based my figure on the Mesulam picture. He calls them olfacto-centric.
There would be different ways to name it, but they tend to project to areas that are closely related to olfactory system. To the amygdala, to the basal forebrain. And they project to areas involved in the-- you could say the limbic tags-- the emotions associated with objects. So always concerned with the object information. Whereas the hippocampus-centric is more concerned with place information.
So that's a way to group cortical regions on the basis of connections. And it's convenient to group the paralimbic areas that way, those transitional areas. These are areas that get a lot of inputs from association areas of the neocortex and they're all connected to deeper lying limbic system structures like the amygdala and the hippocampus.
So whereas within the neocortex now, we see structural differences. The extremes are shown at the two ends in this picture based on von Economo, who, like Brodmann, did a like of cytoarchitectural work on the human brain, as well as other animals.
And he's showing primary sensory at this end. And so just look at layer 4 here, where you see the granule cells. And then look at the other extreme. Primary motor cortex. It's agranular. When you get to the layer 4 region, it doesn't really look much different from the layers above and below it because there's many pyramidal cells and not so many granule cells. So it doesn't form a distinct layer. But that's only in agranular cortex, the motor areas.
And then you have other areas, prefrontal types of cortex, parietal types, and the polar types-- frontal pole, temporal pole, which are a little different. But they still have-- it's always possible to name the six layers. The only places that would be difficult is in the motor cortical areas.
So let's say a little more about these layer 4 extremes. Biggest differences are between the primary visual cortex and the primary motor cortex, where-- so that's what I was just talking about. The layer 4 differences. And we saw area 17 earlier. Well, you see how prominent, even more than in the von Economo picture here, extremely prominent layer 4 is. And it can be subdivided. It's normally subdivided in primates into three sublayers, and then in addition it has those transverse fibers, the line of Gennari.
And we've talked about connectional differences. In this diagram, I take the cell bodies in these different layers and show how the axon from both cell bodies, the prominent direction of axon outflow from those layers-- it's based on this picture which we saw earlier, which shows a slightly expanded view of major connections within one cortical column. It shows the different association types of axons, as well as projection axons.
So it's useful to know these. These represent the thalamalcortical axons, like to a sensory area coming primarily to layer 4, but also layers 1 and 6 and a little bit less to the other layers. It does go to all of the layers, but these are the dense projections. And that densest projection to layer 4, you can see the information goes from layer 4 primarily up by means of the excitatory interneurons, these little stellate cells that have axons that just project towards the [INAUDIBLE] surface and they terminate in layers 2 and 3.
And those layers project heavily to layer 5 in addition to having association connections to other cortical areas I'm not showing those. I'm just showing the intracolumnar connections here. And 5 projects to 6 and 6 and 5 both have some feedback connections to the more superficial layers.
And this-- we used this one before, this picture of a cortical column, to talk about the different types of transverse connections in cortex within the layers. In layer one, in layer 4 primarily. And then transcortically by U-fibers to other areas. I show one here coming from layer 2. Layer 3 cells would have many connections like that also.
And then layers 5 and 6 going to subcortical areas. Corpus striatum, thalamus, brain stem. The ones to the thalamus come from layer 6.
Another way to look at those layer 4 extremes is an interesting one of comparison made by Brian Cragin, electron microscope studies. He just looked at total number of synapses per cell. Just take a little column of cortex, do the same for visual cortex and motor cortex. He did it for rats and for monkeys. And this is for the monkey.
And he counted the synapses and counted the cell bodies, the neuronal cell bodies. He found about 4,000 synapses per neuron in the primary visual cortex. But in area 4, the primary motor cortex, he found 60,000.
What does that indicate? A lot of-- much more convergence in the motor cortex coming from various sources into those neurons in the motor cortex. And I mentioned the different kind-- where those synapses-- the kind of axon that is synapsing on those cells.
I ask here, what are probably the dominant ones in area 17? We always think of the thalamalcorticals for area 17. And, yes, many synapses are like that. But I think you have to ask about those synapses formed by the axons in the line of Gennari, the transverse axons, because they probably contribute large numbers of terminals as well.
Let's look briefly at the functional types and talk about Brodmann's areas. First of all, about functional types. We usually talk about primary sensory, primary motor, association, and limbic areas. I like Mesulam's view, which is very similar.
But he talks about the idiotypic cortex as the most specialized. That's primary sensory and primary motor. There are the areas that are most different from other areas. So that's one type in his nomenclature. Primary sensory or motor.
And then he divides the association cortex unimodal, or primarily unimodal, and the multimodal areas. And then finally, you have the limbic areas.
Now Brodmann used Nissl methods to divide up all of the neocortex and this is the map. And what I did here-- you'd have to enlarge this quite a bit to actually see his numbers. What I've done is I've put red dots by the areas that basically all anatomists know.
The others, we often just talk about the region, like the various temporal regions, prefrontal regions, areas of locus cortex here, and the inferior prefrontal cortex and premotor cortex.
So what are these areas I've marked? Well, 17, 18, and 19 in the visual areas. That is the primary visual cortex, 17. 18 and 19, which are unimodal association areas of the visual system. There are additional ones, but-- because the visual system in primates has expanded so much into the temporal lobe.
And then here in somatosensory. Primary somatosensory areas-- 3, 1, and 2. Those are the Brodmann numbers. And then in the motor cortex, area 4 is the primary motor cortex. And right in front of it, area 6, that's the premotor cortex. These are the areas that get the projections from the ventrolateral nucleus and the ventral anterior nucleus. Area 4 and area 6.
And then people usually know what area 8 is. It's the frontal eye fields. Gets input from visual system. Site of working memory for positions and egocentric positions around our head. We retain the information, at least briefly. It's used in making decisions about where to move our eyes. Makes the saccadic eye movements. Voluntary movements of the eyes. Probably not the involuntary saccades, but the voluntary ones.
So when we come to functional differences, what are the methods that people have used? Just summarize them. What do you remember? What have people done? We're talking about function. They've used--
AUDIENCE: [INAUDIBLE].
PROFESSOR: Sorry?
AUDIENCE: Stimulation.
PROFESSOR: Stimulation and recording. The electrophysiological methods. Those are major methods that have been used a long, long time in neuroscience to specify the function. It does the best for sensory areas. And motor areas they've used more electrical stimulation.
What other method?
AUDIENCE: [INAUDIBLE].
PROFESSOR: Lesions. Exactly. Ablation results. We make them in animals under-- we know where the different areas are in an animal and we specifically try to ablade very specific areas to work out functional differences. In humans it's people that have had strokes or gunshot wounds or other traumatic injury to the cortex.
And that has all contribute-- all of those methods have contributed a lot to our knowing functional differences. And what's the newer method?
AUDIENCE: [INAUDIBLE].
PROFESSOR: The functional imaging methods. PET scanning and magnetic resonance imaging. Primarily magnetic resonance imaging. And now, of course also, magnetoencephalography is used. These are all techniques you can use live people, as well as live animals.
So this is just-- I want to review temporalization briefly, show you one more thing. And then axons to and from neocortex.
We've talked about the big neocortical expansion we call temporalization. If you take a rodent brain here and compare it to these other three branches, you see enormous amount of cortex in the temporal lobe here because this area here has expanded so much. It's just-- it's done that.
So what I did here is I've shown positions of 17 and 18, the oldest visual areas. And then, can you see my-- looks like it's a yellow there. I show this huge expansion of visual cortex there into the temporal lobe going all the way down there. And going in here into the parietal cortex, posterior parietal, down into the-- this is infratemporal gyrus, the infratemporal cortex.
The equivalent areas in the rat, some of them have been found only fairly recently. So here's 17. This they call 18a in the rat or mouse or hamster. It's actually several different areas. So it's actually not a single area.
So it's not totally equivalent, even though it's always pictured that way. It's really not equivalent to area 18 in primates the primates or in the cat because it's multiple areas, all with separate representations of the visual field, not a single one. So that's one difference.
And the other thing is, the area here I've put the orange line around or yellow-orange line is the area-- the only area that we know of in the rodent that's equivalent to this expanded area in the primates. It contains the postrhinal cortex, an area that's really parahippocampal. It projects pretty directly into the hippocampus. And the perirhinal cortex right next to the olfactory cortex. Those are all areas that you do find in these larger brains as well.
Now let's just review something. I want to know about axons coming from somatasensory and motor areas. They go all the way to the spinal cord. These are the longest axons coming out of neocortex, even in animals like a rat or a mouse. You should be able to name them. We've done this before the midterm and you should still remember this.
This is the picture we showed earlier. And I show axons coming out of the cortex, going through the internal capsule, and they become the cerebral peduncle along the sides of the diencepalon in this region.
And then they become the pyramidal tract after passing through the pons. And then they're just called corticospinal tract axons when they're inside the spinal cord. And we also use this name corona radiata. So here when they're coming out of the cortex-- and they have this in a dissection. You can see this radiating pattern of axons. That's the white in the white matter, the thick white matter of the cerebral hemispheres. They're called the corona radiata.
And those funnel into the internal capsule, which is what the axons are called when they're within the corpus striatum or going through the corpus striatum. They're called the internal capsule. They're encapsulated by the corpus striatum.
And then they become-- same axons-- become cerebral peduncle when they're further down going alongside the diencephelon. And then they become-- we can keep calling them peduncle when they're at the base of the midbrain. Then we change the name after they go through the pons.
So what's the difference in the axons of the cerebral peduncle and the axons of the pyramidal tract? What's missing when you get caudal to the pons? Well, all the cortical pontine fibers have terminated and there's huge numbers of them. So it's a lot smaller now.
You could ask the same question about internal capsule versus the peduncle at the base of the midbrain. Well, all the fibers terminating in the tweenbrain are gone now unless they're branches.
So that's all just one of the major axon bundles leading into and out of the mammalian forebrain. What is the other one? This is all lateral forebrain bundle. The other one is the medial forebrain bundle. And these are the pictures we had.
So all of those axons we were just talking about are lateral forebrain bundle axons. They do include some axons from the corpus striatum that don't go anywhere near as far as the ones from cortex. The corpus striatum fibers reach-- the longest ones get to the caudal midbrain. That midbrain locomotor region and midbrain extrapyramidal region. [INAUDIBLE] in the midbrain in the reticular formation.
So these are those pictures that show those two major bundles. And I named the components here. Thought I opened this.
So let's talk more about thalamalcortical organization and transcortical connections and association cortex. And then at the end about thalamic evolution, which we might not get to in class, but it just summarizes for you things that I talk about in the book.
So first of all, if you look at these hemispheres of a rodent, a cat, or a dog-- this is a cat-- and a human, what is the rhinal sulcus? In a rodent it's the one sulcus you can see from the lateral side. That sulcus.
It simply separates the neocortex on one side from the olfactory cortex on the area. Two areas that have very different inputs and different functions. You see it in the cat here as well. You can follow it even in to the temporal lobe.
In the human, it's there all right, but you don't see it in the lateral view because you would have to look from underneath to see it. And in these simplified drawings here of the hemisphere, look at the lower one here. There's the rhinal sulcus.
There is one other sulcus, but it's on the medial side. It's the hippocampal where the infolding of the hippocampus forms a little sulcus there. I'm trying it here sort of the very rostral hippocampus in an animal like an opossum. Other mammals have it there too, but it's much tinier. It's only large caudally.
What does this name mean? Olfactory peduncle. You go to medical school and these terms come up and you'll say, well, why didn't I learn that? Well, look. Here's the ventral view of the human brain. And this is the olfactory bulb.
And then the darker orange there is the actual bulb. But if you insert a spatula under it--and we did this for the sheep, too-- you can actually lift that whole thing out. It's not just the bulb you're lifting out. It's the connections to the olfactory cortex. In fact, there's a little bit of olfactory cortex right in the peduncle.
This all lifts up about back to here. And then the axons continue caudally and they go here and to the olfactory tubercle in the case of the forebrain and then to the various parts of the olfactory cortex. You can look back at the olfactory system chapters ans see these pictures.
So that's what the olfactory tubercle is. And many animals have it. Many fish have the olfactory bulb. It's way, way out in the snout, and the brain's much further back. So you have the peduncle connecting the bulb to the rest of the brain. It's mostly axons.
So we know that those axons of the peduncle are going to olfactory cortex. But they also-- olfactory information does reach the neocortex. How does it get there? It doesn't go directly. That's the first answer. It doesn't go from olfactory bulb to any area of neocortex. It goes to olfactory cortex and closely related structures like the amygdala and the olfactory tubercle.
And there's several parts to the amygdala that's got such direct projections. We're not actually sure about the amygdala projections in humans. But it appears that it's similar human. And hamer has a nice picture of that. Then I based the picture in Chapter 19, picture of human olfactory projections, on the Hamer summary of that work.
So how it does then it get to neocortex? It gets there mainly by way of the thalamus, just like all the other sensory pathways do. Most of the sensory information goes through the thalamic relay.
So what is the olfactory nucleus of the thalamus? Well, there's the medial part of the medial dorsal nucleus which projects to the orbitofrontal context. You can consider it sort of the limbic part of the thalamus. Carries limbic system information to the orbitofrontal cortex. And that does get information from olfactory cortex, as well as other areas. Like it gets input from the hypothalamus as well, and from the amygdala.
But there are other pathways. I probably should have mentioned them in the book, but they're not as prominent. Most of these areas go through the medial dorsal nucleus to get to the orbitofrontal cortex, but the amygdala itself gets olfactory input and the amygdala has projections to the prefrontal cortex as well.
And those parts that get olfactory inputs have projections to the orbitofrontal. And then there are some basal forebrain projections that are getting olfactory input that have some projections that reach that same area.
So let's just summarize now these major thalamalcortical projections. Here's a verbal description of the major areas of thalamus-- the geniculate bodies, the lateral thalamus, the ventral thalamus, both the somatosensory part and the motor part, and then the medidorsal nucleus, the anterior nuclei, and finally the older parts of the thalamus, the intralaminar and midline. And these are the pictures I used. So I've used a color code here and I've outlined in different colors the major regions.
So what is in blue here? The areas that project to what we call central cortex. Central means somatosensory, motor, and premotor. Or just sensory and somatosensory and somatomotor. And probably also somatosensory to begin with, originally. So these are the central areas. They're the areas that give rise to the longest axons that we've talked about earlier, many of which go to the spinal cord from all of these areas.
So I show in the darkest color there the primary somatosensory areas; Brodmann's areas 3, 1, and 2; and this would be area 4 and this would be area 6, the premotor cortex. Those get their inputs primarily from these cell groups in the thalamus of the ventral nucleus. It's hard to see the way I've drawn it here, that these are all more ventral. Actually, the VA does get quite dorsal when go-- that's in the more anterior thalamus. It reaches dorsal surface right next to the anterior nuclei.
So the ventral basal, the most caudal and most ventral part of that goes-- that group of nuclei is getting the direct somatosensory input from spinalthalamic tract and the lemniscus and so forth.
These are the ones that go to motor cortex. Primary motor mainly from VL, which is getting heavy inputs from the cerebellum. Some from the corpus striatum, the globus pallidus. And then the VA, which gets heavy input from the globus pallidus, the striatal outputs. And that's going to the premotor area.
And remember the difference in function between these two areas. Premotor areas stays more involved in actual behavior because they always get patterns in behavior when they simulate there. So simulate the motor cortex, you're getting individual components, single digits, single muscle groups.
There's been a lot of argument about what exactly-- what's represented here. But it's always a small piece of the body.
Anterior nuclei are going to that cortex just above, in front, and behind the corpus callosum. The cingulate cortex and the retrosplenial cortex. And that I've outlined in the pink there.
So now let's what's left? Start with the geniculate bodies. Lateral and medial geniculate bodies, primary visual and primary auditory areas. And depending on how much you include in the medial geniculate, you would include some of the areas around the primary auditory cortex there.
And then what's left? Other than the intralaminar which I don't show here which project quite widely to the cortex. You two major structures. The MD, the medial dorsal nucleus, and the lateral thalamus, which has several components. But some people call-- in primates-- call the whole thing the pulvinar, except, perhaps, for the anterior areas.
Other people like to keep the LP, LV be here, LP, and pulvinar separate. But it's all lateral thalamus, so I grouped it all together and I've shown that that projects to this entire huge expanse in primates. Not so big in other animals, like the rodent. The posterior association cortex, both unimodal and multimodal or heteromodal.
And the MD, same kinds of projections but now they hit prefrontal areas, the areas in front of the motor areas. And I do show here-- you see that I've mixed here with the model pink for the MD projections. I mixed some orange. You see it ventrally here. You see it here.
And then I've mixed some of the model pink there with-- in the temporal pole. That's, quite simply, because these areas-- to the medial part of the mediodorsal and the medial part of the pulvinar-- do have overlapping projections to both these areas. In fact, it's continuous into parts of the cingulate here as well.
So those are the major territories. Obviously there's many different regions that get input from various parts of the lateral thalamus. Same is true for the mediodorsal nucleus.
Now of you take a section from an embryonic human, it looks just like a rat or a hamster or a mouse. There's the geniculate body. That's where the-- you could call it lateral posterior nucleus in a rodent-- but that's what will become the pulvinar as the human goes up. This is an embryonic human.
This is a picture of a cross-section of the very caudal pulvinar. So you would make a section here and this would be a transverse section. It would go right through the two geniculate bodies and through the caudal into the pulvinar, and that's what you're seeing here.
This isn't even the biggest part of the pulvinar. But you see it's grown so much it's pushed the geniculate body over. So you have that horseshoe shape. The medial geniculate is now medial to the lateral geniculate.
And here's the rodent, where I've used the same color scheme to indicate the same kinds of things. You'll note here that the premotor and the prefrontal are not as sharply distinguishable, though they-- by projections you can indicate where the main prefrontal nucleus is just by MD projections. It goes right to the frontal pole, and then on the medial side it covers this whole region.
Colors maybe didn't show that too well, but it's-- also the rostral. This rostral cingulate area, and people vary in how they name those. It could just be called prefrontal cortex. And paraolfactory region down here.
But anyway, those are the equivalent areas in a rodent. And you'll note that the size of the prefrontal areas and the size of the posterior associations are nowhere near the size in large primates, especially in humans.
So Nauta used to say that the association areas are what's left when you specify the sensory functions of the [INAUDIBLE] of the neocortex. But we've learned a lot since that time by functional studies. Now we've expanded the size of the-- it's been quite surprising how many unimodal visual areas there are. They include a lot of this cortex.
So let's just show that here. The best definition now of association areas is in terms of the anatomy. Many psychologists use to think, oh, that should be for-- that's where we form associations, between different modalities and between sensory and motor. But in fact, it's defined anatomically by the long transcortical connections.
It is the multimodal areas that have the longest connection. You can see a lot of them in a brain dissection. This another picture of the major fiber bundles that you can make out in a dissection. And they were given names just on the basis of the dissection.
This unciante transmitted or the hook-shaped bundle from anterior temporal to the orbital prefrontal. And then the arcuate, these fibers that arc around from the posterior parts of the temporal lobe all the way into frontal lobe. And then long longitudinal bundles, superior longitudinal fasiculus, superior occipitofrontal fasiculus.
Now, these have been traced experimentally. Not in humans, of course, although there are better and better methods for seeing these now in humans. But this is a summary that I made up from the various studies that Deepak Pandya and his associates had found. And Kathy Rockland, who has been working with the Tonegawa group for a while, participated in some of these studies.
But this just shows these major transcortical fiber bundles. They're coming from those association areas, primarily the multimodal areas, but also unimodal, here especially for the visual system. You see these many long fiber bundles.
So then I just said, how can you verify these for the human brain other than the use of postmortem dissection? What is the method called? It uses diffusion of water molecules in a primary direction.
So diffusion tensor imaging is a common name for it. It has a couple of other terms that are used and you will find if you just search under diffusion tensor imaging, you'll find many of these studies.
I asked some of the limitations of the method. Main one is resolution. You can't-- you're never anywhere near the level of cells as you are when you do experimental tracing work. And another limitation is it depends on the computer algorithm that you're using to do the tracing.
So the picture I put in the book, if you look carefully, I believe it shows fibers going from cortex up into the cerebellum. Do any fibers do that in any of the experimental studies? No. But they cross, they come right next to fibers of the pontocerebellar projection. So depending on how your algorithm is set, you might get some of these fibers. You would cross from one major bundle to another.
So it's very dependent on the math and on the computational methods you're using. But people are-- they're getting better and better at it. But you always have that limitation of resolution.
This just shows some of the diffusion tensor imaging picture. I think I put this one in the book. It was interesting because it showed a developmental change in fibers that come into the Broca's region of the frontal lobe, areas we know are important for speaking.
And you see in the newborn, they showed two major routes. A third route appeared later. So in the adults, you see three major groups of fibers. You only see two in the babies. So that's the kind of information that we didn't have just on basis of dissections these-- to study things related to Broca's area. Of course, you have to use human brains.
I want you to understand those Mesulam types which we mentioned briefly earlier. And you should know what the association layers of mediocortex are. You can guess what they are. When we talk about association layers, which layers give rise to-- the main layers that give rise to transcortical projections and callosal fibers too? Layers 2 and 3 above-- remember, when neocortex appeared, these new layers appeared. They were superficial layers, layers 2, 3, and 4.
The older cortex, the dorsal cortex of non-mammals, they had the equivalent of the deeper two layers. The association layer, the association fibers, transcortical, come from those superficial layers 2 and 3. So there have been some special studies now of those two layers.
And first of all, I just summarized for you the Mesulam types. I show you this picture, which doesn't show the transcorticals. If you look here under higher order heteromodal association areas, what it's not showing is that these areas interconnect with each other. The posterior association areas interconnect the anterior association areas.
I'm just showing the connections between these areas and the adjoining unimodal areas and the, on the other side, the paralimbic areas. The major route to paralimbic areas is from those multimodal association areas.
And this is a very useful picture of Mesulam. A beautiful color picture that I use the base the functional picture that I drew up for the book showing these major types of functions-- sensory and perceptual, motor, behavior-- meaning pattern movements-- and then motivation. And it's based on the Mesulam depiction of the various types of cortex he shows in this box diagram.
And if you blow it up, you'll see the see the Brodmann numbers listed. He's used-- what do his letters mean? This is M1. What does MA mean? It means motor association. That's what he calls the premotor areas.
So it's unimodal. Still motor. But it's getting many connections from its primary. He doesn't mean it doesn't get any thalamic input. It does. But it also gets a lot more transcortical input. And then prefrontal cortex we see.
So similarly here, the unimodal association provision. But look at the two heteromodal areas. I've left those areas-- the multimodal areas-- white here. So I talk about what are the two heteromodal fields or multimodal fields in the primate brain? Which is larger? And what are the thalamic nuclei that connect with them?
Well, you can see them right away here. The prefrontal area anteriorly. And posteriorly, it's posterior, parietal, and temporal. And much of it is buried in the sulcus here. Those are where the main multimodal areas are.
And it looks like prefrontal is bigger, though there has been argument. And one reason is that the primarily unimodal visual areas occupy so much of the association cortex posteriorly. Here you see them from the medial side.
And what are the thalamic nuclei? Well, the lateral nuclei and the medial dorsal nucleus. Here it is in a rodent and here it is in an embryonic human and in an adult human where you just see the pulvinar there. Maybe a dorsal would still look similar. And there you see the colors showing the relative size in rodent and then in human.
And I won't spend time on Mesulam's different way of dividing up major divisions of cortex. It's rather interesting, but much more difficult to get your head around. And this is the one would be more useful to anyway in the future.
So what we will do next time, I'll say a little bit about Poliakoff and the study he presented done in Moscow of the association layers and the work done in the West that relates to it. The most interesting fact there is that in those association layers, layers 2 and 3, are where, when you look at individual cells and hold them in imaging over a long enough time, you see the cells are changing.
They're plastic. The GABAergic interneurons appear to be the plastic cells of the cortex. And that probably holds true throughout the entire cortical mantle. And more and more physiology studies are supporting that, but we had data from morphology even before the studies I just mentioned, which were done here at MIT by Elly Nedivi working with engineers.
We knew that that's where the growth associated protein remained. It's always present in developing axons, but it remains in the cortex primarily in those association layers, and also in association areas of the thalamus.
I was involved in only a few of those studies with Larry Benowtiz. He did most of it. Rachel Neve, who's working upstairs on the sixth floor, was also involved in those studies.
All right. So we'll come back to that and say a little more about neocortex in the last lecture.