Flash and JavaScript are required for this feature.
Download the track from iTunes U or the Internet Archive.
Description: This lecture is the second of two on the corpus striatum, including discussion of the anatomy, connections and associated diseases.
Instructor: Gerard E. Schneider
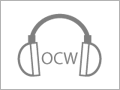
Lec 34: Corpus striatum, pa...
The following content is provided under a Creative Commons license. Your support will help MIT OpenCourseWare continue to offer high quality educational resources for free. To make a donation or view additional materials from hundreds of MIT courses, visit MIT OpenCourseWare at ocw.mit.edu.
PROFESSOR: OK. A little more about the normal striatum, but then we'll spend the rest of time talking about Parkinson's disease and treatment of Parkinson's and-- with methods that are to being explored not just for Parkinson's but other diseases as well.
We ended on this rather difficult network diagram. The one thing that I note that's missing here besides the obvious things like I didn't put in the neocortical connections except the one from the thalamus because it receives a striatal connection. And actually the major output of the globus pallidus is to ventral thalamus.
Where's does the VA of the thalamus project? Does anybody know? You know what the ventral posterior nucleus is. It gets the spinalthalamic tract and the medial meniscus pathway carrying some other sensory information. But that's caudal part of the ventral nucleus. The more anterior parts of the ventral nucleus are related to motor areas of the cortex.
So the VL projects to the primary motor cortex. That's in the middle. And the VA projects the premotor cortex. Both can be considered motor cortex. The VA differs from the VL in that it's more concerned with movement patterns, whereas the motor cortex itself, when stimulated, moves individual muscle groups controlling a single finger, for example. A wrist or elbow or single joint.
But what's missing from this that I wish I had more on was inputs to the subthalamic nucleus, especially because that nucleus is providing the major excitatory projections to the globus pallidus and to the nigra. Obviously very important. If you're missing that the whole system becomes unbalanced and you get some pretty striking movement pathologies in humans.
The only thing I could find was there are projections coming in there, excitatory projections from somatosensory cortical areas and some more directly from somatosensory pathways coming from below. So what exactly that means, what I would like to see is a good computer model of this entire system because that's obviously pretty complex. And I think it would help us a lot in understand how this whole system works.
But anyway, the kind of connections we have here with inhibitory pathways that are then connected to structures that inhibit further structures. So you have inhibition of inhibitory pathways. Obviously important in understanding the kind of things that go wrong with basil ganglia disorders.
Parkinson's disease is the first example here. That's where you get cell loss here in the substantia nigra. And the cells that are being lost are in the Pars compacta here that provides that dopamine input putamen and cuadate. And it leads to-- that's one of the major things that happens in Parkinson's.
And the striatum doesn't work well. You get-- the most obvious symptom is you get a tremor [? regressed ?]. And if they make an intentional movement, they'll stop the tremor. But if they're just sitting still, they'll show this kind of tremor. Very characteristic of Parkinson's disease.
But as the disease progresses, you get an akinsesia. They move less and less. They have a characteristic gate. It's very recognizable.
And then in the late stages of the disease, there are other things that happen too. Cognitive deficiencies show up. Other systems are clearly effected, not just the dopamine pathways and the nigra. But it is that nigral degeneration that accounts for the things that we see when Parkinson's disease is diagnosed.
And other diseases like-- you get cell loss in the striatum in Huntington's disease, which is a genetic disease where it doesn't appear until adulthood. When people-- initially it looks like whenever they intend to move a limb, they, instead of moving the limb in a controlled way, they make a ballistic type movement.
Have any of you ever seen people with these things? Parkinson's disease in aged people is more common. Whether that's really Parkinson's disease, they have Parkinson's like symptoms because they begin losing cells due to vascular insufficiencies.
But anyway, in chorea, or especially in hemiballism-- in hemiballism they have a lesion of the subthalamic nucleus on one side. And when they make-- they intend to make a movement, they make a ballistic movement, that is, a flinging movement.
The claim is that even these become involuntary. To what extent that's really true I'm not sure. But people say they didn't intend to doing that. But on the other hand, we're constantly making small movements. Basically, their habits now cause them problems because they can't-- they don't have normal control.
Huntington's chorea, the genetic disease, they make writhing movements that was named the chorea or dance-like movement. But basically, they're involuntary movements. When people first getting it, they tend to hide it as if pretend they were doing something intentionally. But in fact, it gets worse and worse until it becomes very obvious that they don't have any control.
So we think the double inhibition helps explain it because when you lose a lot of inhibition, cells tend to become hyperactive and produce abnormally increased movement instead of lack of movement. In Parkinson's disease, you get reduced movement except for the tremor. In these others, you get excessive movement.
So let's go back to a more inclusive view of the striatum. Talk about-- integrate the limbic striatum with this. We went through that a little bit in the last part.
The limbic structures are in here in the hemisphere. And we're talking now, in limbic striatum, the structures of the striatum, they give the output of these limbic system structures shown in the red stripes and you know are concerned with motivation and emotion.
And so in-- this is the view we want. If you look at the striatum in the hemisphere, if the hemisphere's transparent, you will see the striatum in these locations. And the entire ventral striatum, I would include amygdala as part of it. We know that the amygdala is actually a combination of pallial and striatal structures, but a good part of it is striatal and it functions in many ways like a striatal.
Like the dorsal striatum does with its connections from neocortex, the ventral striatum and amygdala do for the limbic system, including the formation of learned habits. But the habits, of course, are very different for ventral striatum. They're habits of feeling and emotion.
I don't think I'm going to go through this in detail because, but it's Larry Swansons' more inclusive view of the striatum. I will simply summarize it this way-- he, of course, has first the dorsal striatum and pallidum, somatic system, caudate putamen with its outputs to the pallidum, which are the globus palladis-- two segments.
I just want to point out if any of you deal with rodents and you try to find that internal segment, it's very difficult because they're basically cells embedded in the peduncle. It's called entopeduncular nucleus, the nucleus enclosed by the peduncle. But it is there, nevertheless, because of these cells with the connections. But in the larger animals the pallidum is quite separable.
But all these other structures you could call part of the ventral striatum. But he includes the septal nuclei and he calls the medial striatum and pallidum. And amygdala structures. And he has the pallidal part as a bed nucleus of the stria termanalis.
It's the way he-- some people really like to systematize this and Swanson liked to do that. In my-- I just take all of these structures and call it the ventral striatum because there are a lot of similarities in the way these structures function.
They're all getting outputs. They're all getting out outputs from the limbic cortical regions. They're all involved in habit formation. Pretty much parallel to the way the dorsal striatum works for the non-olfactories and non-limbic systems. All right.
Can anyone answer question eight for me? Just contrast the neocortical projections to the putamen versus the projections to the caudate. How are they different? Why is it when we treat Parkinson's we're always dealing with putamen when actually the caudate is also involved?
AUDIENCE: [INAUDIBLE].
PROFESSOR: Sorry?
AUDIENCE: [INAUDIBLE].
PROFESSOR: It's because-- exactly. The inputs to the putamen are from the sensory motor areas of the neocortex. Somatosensory and motor areas. Areas 3, 1, and 2. Area 4, area 6 in Brodmann's terms.
Why, in question nine-- I'm trying to say here why would you expect the corpus striatum to be involved in almost all of the functions of neocortex? The answer is very simple. Almost all of the neocortex projects to the striatum. You can say a similar thing for the cerebellum. All of those structures, they get cerebellar input. Input from neocortical-- so many neocortical areas project to the pons, which then projects to the cerebellum.
So we know that the cerebellum then has got to be involved in more than just simple coordination of movement. Because there's a coordination of a lot of things involved with timing adjustments that the cerebellum is so good at. In this case, it's the striatum.
That means when you have corpus striatum pathologies you can get many different problems besides-- but the thing that people notice the most, the most common complaint of the people with damage to the corpus striatum, or of course any of these diseases, is the effects on movement.
This is just a picture shows the central cortex here. And by central cortex, we always mean somatosensory, motor, and premotor or supplementary motor areas. There's a number of premotor areas or supplementary motor areas. One of [INAUDIBLE].
And it shows the projections from there in the reddish brown going to the putamen lateral to the cerebral peduncle. This is lateral to the internal capsule, I should say. And the internal capsule fits in here. The caudate nucleus is mostly medial to it. The anterior-most part is the largest [INAUDIBLE] caudate.
It's getting inputs from all of the other cortical areas, including some of these areas [INAUDIBLE]. This is from a picture in [INAUDIBLE]. And we leave some of these white, including the visual areas here. But in fact, even in a hamster that I've studied I've traced projections here from the visual area as I see the projections of the caudate nucleus. And it also includes prefrontal areas here.
All right. And this is an updated view of the projections from the cortex. And they just show one section of the cortex here. How it projects to the entire striatum, both dorsal and ventral, and also to the intalaminar and midline nuclei. Because these nuclei, remember, have direct projections to the striatum.
So that means this little area here, the [? pair of them ?] [? trick ?] to the nucleus around the midline nuclei. It projects to this corner of the striatal structures, including where this basalis is.
And this area of cortex projects to that same area. So you'll see it's a highly organized system. The parts most similar to dorsal-- what we've been calling dorsal striatum involved getting the input from somatosensory and motor cortex. They show in green here. You can see here that that's going to the dorsal lateral region of the striatum.
So the ventral striatum is really ventromedial here. Dorsolateral then ventromedial according to more recent studies of these projections.
And the input to the caudate is much more complex. I went through some of the studies and found this kind of system where you got projections from orbital frontal cortex that form these huge columns medially. And they can be traced in that same position all around to the very tail of the caudate. This is internal capsule in here.
And here are other parts of the dorsolateral prefrontal cortex. Posterior parietal, which is very far from the prefrontal. See, it's all these different areas here and here are projecting this complex pattern to the caudate. But it's actually more complex than that because if you look at the actual studies like here, they trace projections here from prefrontal areas and here from parietal cortex.
And then you look at a part of the striatum. You see these-- it's not entirely blue here and pink here, but in the middle here there's a lot of interdigitization indicating that there's a lot we don't understand about how this is working. That is, the striatum itself must be doing some integration of these inputs because the interdigitization of the projections of the anterior and posterior association areas-- this area and this area. Those two projections tend to be very close to each other in the striatum.
Why is that? To do that we have to understand a lot more about that cortex, which we haven't talked so much about yet. But in fact, this pattern is not well understood. It's functions are not well understood.
This slide is just talking about-- in clinical neurology they talk about these different functional circuits involving the striatum. All of that's to be expected when you know that all of these different cortical areas project there. For example, frontal eye fields of the frontal lobe we know are critical for intentional eye movements can be affected by striatal lesions.
Well, that area projects to the striatum, just like the motor cortex and the somatosensory cortex do. Other parts of the prefrontal cortex project there and, because of that, other cognitive functions that are so important in human functioning that depend on prefrontal cortex, you'd expect them to be damaged. It's just not as common in these basal ganglia diseases. Maybe it's because we don't know.
A lot of people go through life without actually exercising higher cognitive functions that well. I saw that with tongue in cheek, but I think it's probably right. So we don't notice when they suffer a pathology. We just think he had too much to drink a little bit too often or he's getting old or this or that. Whereas in fact, it can be due to these striatal functions.
Similarly, the limbic areas affect movement and motion because they project to striatal as well. Striatal damage or any pathologies to the striatum, that's a common area for interruption in vascular supply to the fore brain. Vessels that come in from the base of the brain go up through the ventral striatum and sometimes they reach the internal capsule as well. So you'll get not just the movement disorders from the interruption of those axons of the internal capsule. You'll get changes in mood and emotion as well.
Another complication that I mentioned in the chapter is the stria zones. This is an interesting compartmentalization. It's a little different from that interdigitization of the projections from the anterior and posterior association areas of cortex.
This refers to the projections of acetylcholine axons and the staining [? for ?] cholinesterase, that you get in the striatum. There are areas in the striatum where there's almost no staining with cholinesterase and other areas where there's heavy staining. We call these p-zones where there's no staining.
We know they have to be functionally different. It's been very difficult to work out the functional differences. I mentioned some of the recent work in that area [INAUDIBLE]. I think we talked about the-- oh, these are about the cell types. There's huge numbers, but most of them are characterized.
These are just structural divisions where you have these large interneurons of the striatum. This is throughout the striatum you get these cell types. Acetycholine-containing cells that-- they're short axon interneurons using acetyocholine.
So we know that that's why there's cholinesterase staining. And yet, now we know there's these areas that don't get it. We just don't know completely why that's true. And there's many cells that use GABA. There has to be because of all these inhibitory projections. We know that the dorsal striatum projects directly to the nigra with a GABA pathway. It projects to globus pallidus, both segments, with GABAergic pathways.
But those GABA cells, the GABAergic cells, can be further characterized in terms of peptides they contain like one type contains not only GABA but substance P, and it tends to project to the nigra and to the internal segment of the globus pallidus. GABA with [? encephalon, ?] those cells project to the outer segment of the globus pallidus.
So they're using the same main neurotransmitter but they're modulated by different peptides. Again, not fully understood. But clearly they have some functional difference.
This is where I mentioned the stria zones and those acetylcholine internuerons. I just want to say a little bit about these terms used for humans. The lentiform nucleus. Did I talk about that before?
This shape right here is the lentiform nucleus, the lens-shaped nucleus. The outer part is putamen and the inner parts are the two segments of the globus pallidus, the output structures. That's the lentiform nucleus.
There's the internal capsule, and medial to it there's the caudate nucleus. It looks small here, but this a big human brain so it's actually not so small. So you see the same thing on the two sides.
I think we saw this before when we talked about the output, the [INAUDIBLE] [? to the ?] [? meticularus ?], the pathway that goes from the globus pallidus here that loops around the internal capsule and goes to the ventral anterior thalamus.
OK, so you can see if you were doing a dissection how you would get to it and go into the sylvian fissure here. Here it is in the photograph. You can just push it apart. You'll expose this buried cortex. What is the buried cortex called?
It's called the island. The insula, the island cortex. Sort of a-- I guess it looked like that to anatomists going in through the fissure and finding a cortex down there that's covering the outer part of the striatum here, the putamen.
There's another little layer of cells there between the putamen and the neocortex called the colostrum. It's very widespread inputs and I'm not spending time on that in the class now.
But we talked about temporalization and how that forms. I just wanted to point out that in this picture these are the axons that form the internal capsule coming down through the striatum. So this is left hemisphere. The caudate nucleus is on the other side of it. It would go all the way around.
But back here what does it do? Well, the caudate goes all the way around. So down here you see it below those axons. So if you make a cross-section, you can see the caudate there and there.
There's white matter in between the caudate and the other parts of the putamen. And here we see white matter here between cuadate and putamen. [? And ?] the whole length of [? that nucleus ?] in fact.
And remember that if we follow the caudate all the way around right here it abuts the amygdala. So this would be the position of the amygdala here.
So let's talk about Parkinson's disease now. I'm sorry I didn't deal with-- I left a lot of things hanging there, but it represents the way the field is to tell you the truth. There's a lot we don't know.
So I want to talk about one of the major treatments that was developed in recent years. It's interesting because it was based on neuroscientific study that began with studies of rats and then moved to a new treatment for Parkinson's disease using tissue transplantation from fetal humans, the product of abortions. They're obtained in abortions. Tissue that would normally be thrown out, but they were legally able to get that tissue and use some. You will see about how old they had to be, but they were always from tissue that would have simply died if it wasn't used.
But then we'll talk about new neurons for the adult brain. Not just implanted, but neurons that are either from stem cells or normally generated in the brain of adult animals and mammals, including humans.
We walked about that once before when we talked about the olfactory bulb. I talked about new neurons being generated in the lateral ventricles even in adulthood and how they migrate forward into the granular layer of the olfactory bulb where there's cell turnover. Constantly those cells in the olfactory bulb are being replaced. The same thing happens in the hippocampus so we'll talk about that.
So let's talk first about the fetal nigral transplantations, a therapy for Parkinson's disease. [? High ?] brain imaging is used to assess the success of those transplants. Before any functional effects are seen, they can find out if the cells are surviving. And then we'll talk about the new cells.
Does everybody understand what Parkinson's disease is? Why is it that-- we know the cells of the nigra that produce dopamine are gradually lost in Parkinson's disease. Why is it they don't see any symptoms of Parkinson's disease until 80% of those cells are gone?
Any suggestions? You remember what I said in the book? Why don't you see symptoms until 80% of them are gone? In fact, as we will see, the treatments for it would be more successful if they could catch it earlier. But they don't.
The reason seems to be collateral sprouting. When you remove a small proportion of the dopamine-containing cells, the axons die going to striatum, the dopamine axons. But the remaining ones simply sprout collaterals, take over the space. There's a lot of evidence for that because it happens in many systems, not just in baby animals but in adult animals. You get collateral sprouting into denevated areas.
So for example, if I cut-- remember I gave this example a long time ago, Chapter 13. You lose axons in your hand due to an arm injury or maybe pressure too long on your arm transects some axons. They will regrow, before they regrow, you can lose function. You can lose your ability to extend your hand, for example. I mentioned that that happened to me once.
And then what happens? You don't have to wait for the regeneration to occur before you get return of function. Why is that? Collateral sprouting, because you haven't killed all the axons, the remaining ones will sprout over a period of about two weeks. That will happen and you start getting most of your function back.
You have to have adequate innervation or you feel extremely weak. You have to have a lot of innervation to get those muscles to discharge properly.
But the same things happening in the corpus striatum. Collateral sprouting. And because it's ongoing, it's probably the reason why making lesions gradually, as the skin a slow-growing tumor. It's also a lot harder to detect because you're getting connections being replaced. It's when you make the lesion all at once that you see the maximum effects of the lesion.
Anyway, the most dramatic discovery of a treatment concerns this use of tissue from fetal nigra. And this is what I mainly what I want to talk about here, fetal nigra transplantation.
They're basically taking nigral tissue just removed from a fetus. It's little chunks of tissue but in a solution. They suck them into a pipette and inject them. That's basically what they're doing.
Why do you think it might be true that the topographic organization of connections isn't a critical factor in the functioning of the nigral striatal connections? You're not causing a regeneration of nigra to striatum. You're just putting the nigral tissue directly into the striatum.
So normally the striatum doesn't contain dopamine cells. But if they put the dopamine cells into the midbrain, do you think the axons are ever going to reach the striatum? It's a big distance in an adult human. It wouldn't work. Yes?
AUDIENCE: [INAUDIBLE].
PROFESSOR: Right. That's part of the research that was done.
AUDIENCE: [INAUDIBLE].
PROFESSOR: No. No, but they were interested in working out the best ages of the human tissue because you don't want to go doing that procedure on humans when the fetal tissue is not in plentiful supply, especially because then putamen-- they're putting it all in the putamen because they're trying to treat these bad motor symptoms, you see. So they're not focused on caudate nucleus. They're focused on the putamen.
And the putamen is very large in adult humans. And those fetuses are very tiny. So to get-- the problem is you put it in the putamen, the axons from those new cells that they're putting in there don't grow very far. They grow very slowly and they don't grow very far. We'll review that quickly here.
So you need a number of fetuses to get a really adequate implant for one patient. And that means it's hard to get enough fetuses.
AUDIENCE: [INAUDIBLE].
PROFESSOR: Because there's a lot of people with Parkinson's.
AUDIENCE: [INAUDIBLE].
PROFESSOR: Yeah. Even if they could do that-- and I don't know of any research that really tried that. But in other systems they have tried, and generally it fails because once systems contain a lot of myelin, the myelin inhibits the growth of axons because of [? the nodal ?] protein. It's very difficult to get it to grow. [? If ?] maybe a prior knowledge of how to get it to happen were a little more advanced, we could create pathways where it would go.
You see, the problem is you're putting the dopamine cells directly into the putamen. That means the normal circuitry, the normal stimulus for nigral cells is missing. But what they were counting on is-- and this worked in rat research. They got a lot of function back in spite of all that.
OK. So if you don't think the topography is very important because we're just dealing with a-- as I point out here, the actual dopamine release doesn't require high information content. It's a neuromodulator. It's simply changing the way those cells are working in the putamen. So that's why we think. It really doesn't matter the topography [? for it. ?]
AUDIENCE: [INAUDIBLE].
PROFESSOR: Yeah, well, that's another issue, isn't it? Stria zones versus non-stria zones could drastically affect how it's working, at least subtle aspects of movement and the way people learn habits and so forth. Maybe they can't learn much. You'd like to look to see how much do they really study these patients that do get some recovery.
But the question you're asking is a major one. You need to find those things out.
We know that the donor age is critical. And why would we expect that? Well, studies of the development in, ontogeny of human dopamine producing cells, indicate that they first appear five and a half to six and a half weeks post-conception. And little processes grow out from those cells beginning at eight weeks post-conception. And the axons first reach the striatum at nine weeks.
Well, once they've already grown all the way to the striatum, we already know from various studies of animals that when you damage cells that have already grown a lot of processes, that they become much more vulnerable. They're going to die if you damage them very much. Because then you're damaging a lot of processes.
So it was predicted that you would have to go in this early period before most of those process, the dendrites and axons, have even grown. And that's what they found. And to do that research, as you mentioned they're actually taking the human tissue and putting it in rats striatum when they found out that they could do that successfully.
They wanted to find out which ages to use. So here they took-- you see a lot of surviving cells. The black indicates the dopamine. Actually it's tyrosine hydroxylase, the enzyme that you always find in dopamine processes themselves. They took it from a fetus 41 days post-conception and it survived very well in the rat striatum.
If they took from 72 day post-conception, that's after the axons have normally already started reaching the striatum, then they didn't get good survival. So they knew they had what-- that's how they found out the age range they had to use.
Now how can they use imaging of the living brain to assess transplant success? By using fluorodopa, which allows you to use positron emission tomography and find evidence for living dopamine cells.
And of course if the patient dies, they can use nueroanatomical methods. Basically [? indianal ?] staining for tyrosine hydroxylase. And they can assess then post-mortum.
OK. You can read about fluorodopa online and how it's [? the-- ?] and this just mentions histological procedure on the autopsy material to find out where those dopamine cells are. And this is just some of the results. The dark staining here is dopamine. And you don't get tyrosine hydroxylase unless it's in live cells.
And you see, if you look in higher magnifications, the whole feltwork of processes dopamine has-- this is all from a graft because when they do these-- this is from a human brain with surviving graft of fetal tissue. There's a lot of dopamine axons in parts of the putamen.
If they get a long ways from where they put the implants in, this is what it looks like. Almost nothing. On the right. Because they don't [? grow ?] very far.
Here's where they're putting in rat and you see very good survival here. And here you see them surviving, how they use to predict when they would to be successful in humans.
So-- and I mentioned this. It makes a big difference on where you put it because of the size. The striatum is so big. This is about the rate of growth. I used six here to calculate that they would grow about a millimeter in seven days at six microns an hour.
And we know that they never seem to grow more than about two and a half millimeters. Because remember, this is adult tissue and there's a lot of inhibitory factors. It's surprising to some people that they grow even that far.
OK, so we know the locus is important because of the functions of the striatum. They occur in different parts and they're interested in the motor symptoms so they put it in the putamen.
So why do you think it's so slow? It takes many months, even longer than the axons are grown out, according to [INAUDIBLE] results. So we know that there's probably other aspects of maturation of the neurons require a lot of time.
So it took a lot of patience in doing this. And I mentioned other issues that are involved. Lack of normal inputs, the fact that Parkinson's disease involves other cell groups than just the dopamine cells. It involved norepinephrine cells, for example.
We know less of about the function of those groups in the striatum. Then I mentioned collateral sprouting in addition. The point is if the dopamine axons are showing collateral sprouting, well, what about other axons? When there's denervation, other axons can show collateral sprouting, too.
So then the dopamine axons have to compete with those. And the competition can take a very long time. And it might not always be successful. It's not easy to predict which axon [? is moving ?] out. Usually the normal axon is [? moving ?] out. But now they're not from the same person. They came from elsewhere. Does that mean they can compete just as well as the person's own dopamine cells would? We don't know.
So I'm asking about alternatives for that treatment. I think I won't take time to go through this. We're going to read about it. The stem cells are the most interesting.
Scientific studies indicate that for advanced Parkinson's patients there doesn't appear to be much use of using this procedure. It just doesn't work. It worked well, though, for people that are less severe. That is, the Parkinson's hasn't progressed as far. So for them, it did work.
But of course, they're really concerned with those people with the more advanced symptoms. And they decided that the transplant procedure just wasn't good enough, so that led to other types of treatments.
The thing used in the clinic-- there's two methods used in the clinic. One is called deep brain stimulation. If you go online and just type in deep brain stimulation, you'll find all kinds of articles because residents-- medical residents in hospitals love to do research and get their names on papers, so you'll find paper after paper after paper on their use of deep brain stimulation.
And yes, it works very well. You stimulate the globus pallidus. You stimulate the subthalamic nucleus. You can get effects. Often you can benefit the patients. Which remember, they're missing a lot of the normal input from those pathways that affect the system. So if you stimulate it artificially, you can improve their symptoms and that's what they [? found. ?]
They also have gone in and just ablated structures. And if they do that right-- the problem here is predicting. Well, how do you know? You only get one chance. Ablated, you're not going to get a second change.
But they have found specific lesions that-- they base it on animal work first. And they do get some benefits, reducing movement problems. And I just mentioned examples of lesion.
I'll talk a little more about the neurogenesis things, but make sure you read that here and I'll answer any questions you have about it. There's a few very interesting issues here about using-- both in using stem cells, which-- if you just look now, do a search on stem cell treatment for Parkinson's, you'll see there's a lot of recent things.
Some of it's by patented cell lines, which I think is a horrible idea that the government has allowed it. And so now that creates all the problems that patents caused and a lot of competition between groups and so forth. But they are getting stem cells to work, but it's not an easy thing. There's many issues in getting those cells to do what you want.
And the-- but I want to say a little bit about the normal stem cells we have, creating new neurons. In the hippocampal formation, not just in the olfactory bulbs. So we'll say a little bit about that because we've talked about hippocampus before.
And then the main topic for next time will be neocortex. First chapter on neocortex, Chapter 32. And I posted questions on that chapter. I've written questions for the next one too. I'll get those posted probably later today.