Flash and JavaScript are required for this feature.
Download the track from iTunes U or the Internet Archive.
Description: This lecture is the third of five on the hypothalamus and limbic system and covers the core pathways and anatomy.
Instructor: Gerard E. Schneider
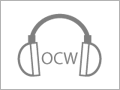
Lec 29: Hypothalamus and li...
The following content is provided under a Creative Commons license. Your support will help MIT OpenCourseWare continue to offer high-quality educational resources for free. To make a donation or view additional materials from hundreds of MIT courses, visit MIT OpenCourseWare at ocw.mit.edu.
PROFESSOR: OK, instead of a quiz I'm trying to write some homework. And some of them will be extra credit, some of them will be part of the required homework. Now today we still have a lot to do on chapter 26 concerning core pathways of the limbic system. And this is where we finished last time. We may not even get to the part on hormones and brain development and effects on the brain, but we may just do most of that with the reading because I really need the time to talk to you about the recent changes in understanding of the hippocampus and the pathways related to it.
OK, we answered this question with the information about the internal environment in the body reaches the hypothalamus via both the bloodstream and sensory pathways. And then I asked this about, well, how do these molecules even get in the brain? We know that there's a blood-brain barrier. Very small molecules can get in, but not the larger, organic molecules.
And yet, things like angiotensin II do get in, but they get in only at certain places. Places where the blood-brain barrier is weak. I think the figure in the book is a little better than this one, but this is a similar one.
It just shows the site from the walls of the third and fourth ventricles where the brain barrier is lacking, and the ones you hear about here, mainly we talked very early about the area postrema where toxins in the blood can get in and elicit vomiting. The subfornical organ has a weak blood-brain barrier, and the median eminence of the hypothalamus. And that's where I want to look now just to show you an experiment on the blood-brain barrier.
This is where, with injection of horseradish peroxidase-- which is a tracer because it's transported both anterogradely and retrogradely in axons-- but if you just put it in the bloodstream, not in the brain, it doesn't get in the brain everywhere, just where the blood-brain barrier is weak. And so here we are at the base of the third ventricle. So we're in the hypothalamic region. This region is called the median eminence. It's near the stalk of the pituitary.
And most of the hypothalamus here and, of course, thalamus and other areas that you can't see here, you see the HRP in the blood. So it's in the capillaries. These are all little capillaries in some larger vessels. It's always, with this particular method, it's showing us bright white. But look at the median eminence.
The HRP pervades the tissue in the median eminence. And that's the kind of experiments that have been done now in a number of different animals to show these areas. And in those areas there's always receptors-- in neurons, generally-- that respond to specific things-- like angiotensin, for example, and other things. Here in the median eminence you would have sexual hormones, for example, secreted by the gonads that can affect the brain by getting in this route.
Now what about sensory inputs? Well, we know the vagus nerve carries inputs about pain, temperature, stomach distention, other visceral inputs. And there's also inputs coming from the sacral region, including inputs from the genital region and basically the lower viscera. But there are other pathways that just come in through the dorsal roots. Pathways carrying pain information, for example.
And this is an interesting one because pain information can reach the hypothalamus directly from secondary sensory neurons and tertiary sensory neurons in the spinal cord. So it comes directly from dorsal horn. There are long pathways that do reach the hypothalamus. It's the lemniscal pathway that I don't think I mentioned-- it's not a real large one but it does exist, and could be very important in responses to pain. We know that pain elicits activation of the sympathetic nervous system and results in inhibition of most parasympathetic functions, and it does so very quickly through this pathway.
OK, then there's inputs from neurons in the hypothalamus that respond to temperature, or to the osmolarity of the blood. If you record for most neurons-- I may have mentioned this when we talked about regulation of the internal environment before-- but these neurons change their firing in very precise ways with the temperature. It's obviously pretty important for the brain to get that information and it's going to control temperature regulating mechanisms. They're in the anterior hypothalamic region.
And then we know there's some olfactory inputs that reach pretty directly. In fact, many more animals, the olfactory bulbs project directly into the hypothalamus. But they project indirectly there in all the vertebrates. And we know the retina projects directly to the hypothalamus. Mainly to the suprachiasmatic nucleus where we have the biological clock mechanism, but there's other regions too that get sparser inputs from the retina. We talked about that in chapter 20.
OK, now I want to start talking about some of the circuitry that links the hypothalamus to endbrain structures, the limbic endbrain structures. And these studies were given a lot of impetus by James Papez, a neuroanatomist working at Cornell in the 1930s. And there's been a big revival of interest in that circuit. I mean, there are a few neuroanatomy texts for medical schools that don't even mention James Papez anymore, but that's because they're not keeping up with all the recent interest in and research labs in some of these pathways. So I want to go through them.
So we talk about Papez circuit, which is interesting because it's a complete circuit. You can start at any point, but if you go to the cingulate cortex, we know that cingulate is one of the paralimbic areas that sends projections to hippocampal, parahippocampal regions, which then project directly into the hippocampus. And the hippocampus then projects to subcortical regions through the fornix. A major output. Not the only output, but it's the major output going subcortically.
It goes to the septal area, but it continues. Long axons continue right to the hypothalamus. Most of them terminate in the mammillary bodies at the very caudal end of the hypothalamus. And those structures project to the thalamus-- the anterior nuclei-- which connects to cingulate cortex. So it forms, then, a complete loop.
So Papez published this paper in 1937. It was called "The Proposed Mechanism of Emotion." And basically he argued that people had associated a group of structures in the forebrain-- especially the endbrain-- with olfaction. And he said they weren't really olfactory in humans because the clinical information-- and he reviewed a lot of it-- indicates that they're really involved in emotion, emotional expression, emotional disturbances, and the feelings accompanying emotions.
And he also noted one other thing that people often forget about. They have a low threshold for seizures, so seizures that tend to start in one of these structures will spread through all of them because they're closely interconnected, which was one of his arguments. Remember, he didn't have the precise neuroanatomical methods that we have now.
And believe me, diffusion tensor imaging is not going to give you these connections adequately. It'll tell you the main tracks, but it's not useful for this. You need good anatomical techniques where you can use animals to do experiments and trace connections to synaptic endings. But Papez was remarkably accurate in his basic description of the circuit based on clinical work.
But now we can take that original circuit of his and expand it a little bit from more recent studies using experimental neuroanatomical techniques of the Nauta type, OK? And many people that are followed now with various kinds of tract tracing techniques. I should also point out here that it was during this time after Papez that the term "limbic system" was coined, based on Broca's term, the great limbic lobe.
In modern times, it was Paul MacLean at the National Institute of Health, a colleague of Nauta's, that actually coined that term, the limbic system. And Nauta then extended it into the midbrain, and did a number of studies of connections of that system in the endbrain. But I'll focus on the way our understanding of the system has changed. It won't sound like we're discussing mechanisms of emotion for the most part, but we will get to that also.
OK. The reason there's such a revival of interest, of course, is because of the involvement of the hippocampus in long-term memory formation and what we call episodic memory-- not habit formation, not striatal memory which we've mentioned a number of times, but the kind of memory that we usually mean when we say, I remember something. Yes, I remember something, something happened. I remember something that happened over there. That's what we're talking about here.
And that certainly adds to the importance of understanding these circuits and how they work. Now if you look at a medical school text, you're likely to see something like this. Just to show you, it can be really difficult because they only give you human brain. So I'm not going to do that. I won't expect you to understand it once you begin to understand the circuitry.
So we're going to take our diagram here of the mammalian brain. Let's take this one and turn it around like this. And now let's just slice off the hemispheres like this. OK, we'll throw this one away, and turn this on its side so we get this. Here's the hemisphere up here, and here's the brainstem down here.
We're looking at it from the side showing where a few of the pathways are. So we've made the brain transparent. And the same here in the hemisphere where I've shown the hippocampus there. And now I want to know how-- this was the original figure here-- I want to show you where you get Papez circuit in that. Very straightforward.
OK? So let's start this time with the mammillary bodies. Here we are at the caudal end of the hypothalamus. The mammillary bodies project by a very direct route. We always see this in cross sections here in the diencephalon.
Trace the mammillothalamic tract to the anterior nucelei. There's three main nuclei that project to various parts of the cingulate cortex. They follow a route to the cortex through what we call the anterior thalamic radiations. They don't go out the side like, say, the geniculate bodies do.
They go forward and then course just above the corpus collosum, throughout the entire length of the corpus collosum, terminating in the cingulate cortex at all these different levels. And terminating also in the area just behind the collosum in an area we call the retrosplenial area, meaning behind the splenium of the corpus callosum. That's the caudal end of the corpus collosum.
Anterior end is the genu, the caudal end is the splenium because of its shape in humans. And cingulate and these other parahippocampal areas, including the entorhinal cortex which gets heavy input from the cingulate and retrosplenial areas. They project directly into the hippocampal formation. And then here's the output of the hippocampus, through this thick column of fibers that courses just in through the caudal end of the septum in front of the thalamus.
And then, because I've separated it here, I pick it up again right here. There's the fornix columns coming through the hypothalamus. There are some axons that terminate on the way, but most of them are ending there on the mammillary bodies.
OK. So now let's take that circuit and see how-- I mean, just the circuit, what good is that? You've got to know, well, what's coming into it, what's coming out of it, and what purpose, what kind of information does it carry? And there, initially anatomy was way ahead of the physiology. Within the anatomy was done well enough that physiologists had better guides and they started doing different kinds of study.
So I'm going to talk about some physiology here. OK, so here's a diagram. A more up-to-date version of Papez circuit. And I've shown in red those structures that Papez named for human-- mammillary bodies, anterior nuclei, cingulate cortex, the hippocampal formation. These are the major components.
Subiculum and hippocampus are one line of cells, and the dentate gyrus. And then we're going to be looking at that very soon in a little more detail. And then there's that output, the fornix. And notice that there's a less direct route that goes through the septal area to the hypothalamus as well. So I've included all that here, and I've included some accents that go in the reverse direction.
And I've shown that many of these, in fact, are two-way connections. As like most cortex, it gets input from the thalamus. Those cortical areas project back to the thalamus too.
OK, and I ask here in this question how's it connected to neocortical areas. Well, look right here. See, this wasn't discussed so much by Papez, but we know that these cingulate areas and other paralimbic areas-- like the entorhinal area-- are closely connected to neocortex.
They get major inputs from association areas, not from primary sensory areas. They're not getting it from visual cortex or auditory cortex or somatosensory cortex. They're getting it from association areas, particularly from the multimodal association areas. OK?
It's an interesting thing, those multimodal association areas are like the most primitive cortex, but they are the areas that have expanded the most in recent evolution. And they're getting major inputs from the unimodal sensory areas. They get their input from primary sensory areas.
OK. So the association areas then connect into Papez circuit through these routes I'm showing-- through cingulate, retrosplenial, and entorhinal areas-- which then project directly into the cortex. There's more than one route there, I just indicate a couple of them.
OK, so what is going on this route here? What is the mammillary body telling the cingulate cortex and hippocampus? It concerns our sense of direction.
And it's a big surprise for many people because we were thinking all the time in terms of Papez and emotion, all this, but you'll see how, in fact, it doesn't [INAUDIBLE]. Where does our sense of direction in the world come from? How do you know where you are?
So you're taking a walk. It's a little harder to think of when you're inside a building, but really not that much. But think of an animal walking. He always has a sense of direction. When he doesn't, we say he's disoriented.
When we feel very disoriented, well, what happens? When we lose the landmarks we were depending on? And for primates and many other animals, it's primarily visual. Even a rat-- not such a visual animal, but yet his major landmarks are still visual. That's how he knows where he is.
And that's usually detected by cortex, a neocortex. So we know what direction we're going with respect to landmarks, even when we're not really aware of which landmarks we're using. OK?
That head direction, with respect to landmarks, is you can call it allocentric head direction. If I want to know where that object is with respect to my head, that's not what I'm talking about here. That's egocentric.
That has to do with where it is with respect to my head and eyes. Now I'm talking about where we are with respect to some landmark. I know where I am here with respect to the doors, with respect to the status center, things like that.
That's allocentric head direction. But that's changing all the time, I'm moving my head around. An animal is moving his head around. OK, he's got a wonderful system for taking those changes in head direction and affecting the activity that's being sent by hippocampus down to the mammillary bodies, and correcting it for changes in head direction. That's what's happening there in the mammillary bodies.
So from moment to moment, the signal going from mammillary bodies back to the cingulate and hippocampus is always corrected for the momentary head direction. So it's constantly changing. That's why it's so important. Why is that so important? Because the way my head is pointed is, potentially, where I can go.
Think of an animal foraging. He can turn this way and that. What is important for him to know? What's important for him to know is what's in front of him. And he remembers things that are in front of him.
He has a map of the environment that he's learned. So all the time, the hippocampus is connected through the cortex and is activating these memories of things that happened in those areas. So he knows, am I going towards a good area or a bad area?
We know, are we going towards food or not food? Are we going towards our friends or not towards our friends? Are we going to our classroom or not? So you begin to see the connection of the circuit with memory now.
So how does that change in head direction work? Let's go back to the Papez circuit here. Well, here's the mammillary bodies and they're getting input from these structures-- the caudal end of the midbrain. Some books say the rostral hindbrain, but they're right at the caudal end of the midbrain in most books.
OK, then the tegmental nuclei of Gudden. OK. And they carry information derived mainly from the vestibular sense-- probably from proprioception as well-- about any change in the direction of the head. All right.
You can record head directions and you find them parallel on that circuit. So the information they're carrying is always is corrected; it's changing every time the animal moves its head. Well, there's other ways. We know we've moved our head not just from vestibular sense; we also know it from vision. So I put in here-- lateral shifts in head direction do excite cells in the pretectal area.
OK. And there's a pathway from the pretectal area that goes to this nucleus, the lateral dorsal nucleus which sits right next to the anterior nuclei. So I put that in here because they're carrying head direction information to the lateral dorsal nucleus, and it's a system completely parallel to the mammillary body to anterior nucleus pathway. So you're getting changes in head direction coming into the lateral dorsal nucleus which also gets projections from hippocampal formation.
So in both places, you're constantly correcting that information about where we are with respect to landmarks. We're correcting it for changes in head direction. I know this makes it seem a little more complicated, but this is the simpler diagram here. You can just remember there's a parallel pathway there for visual information.
I want to point out that the axons in Papez circuit are more than one type. And the ones signaling head direction are the ones that physiologists have characterized the best. We often don't know what the others do, but it's a large number. A lot of the axons in the mammillothalamic tract are involved in this. I don't want to give you the sense that we know everything about it, but we do know about a major component of it.
We also know the hippocampus-- through the fornix-- is sending information to other areas than just the mammillary bodies. It may be altering motivations, motivational level, according to what you're facing. We know it more from what it might be doing from behavioral work than we do from anatomical and physiological work. OK.
A few other terms here-- you should know what the basal forebrain is. And if I go back to this picture-- when you talk about basal forebrain, if you look at the bottom one here, here's hypothalamus. It's a little bit arbitrary where hypothalamus ends here. We say it ends about-- if this is the optic chiasm, where the optic tract is crossing, the areas in front of the optic chiasm are basal forebrain.
If you look at sections-- let's look at these sections for a minute-- and start back here with things we've studied before. Here's the midbrain section, there's the central gray, and the ventral tegmental area, goes to the limbic midbrain areas. When you follow these forward, both central gray and ventral tegmental area are continuous with hypothalamus. You follow either of them forward and you come right into hypothalamus, posterior hypothalamus.
So here we are in the middle of the hypothalamus, forming the ventral part of the tween brain. Here we are a little further forward. You see the optic tract now just behind the chiasm. The fornix fibers are a bundle at the medial edge of the medial forebrain bundle there, which basically courses through the whole lateral hypothalamus.
But then you go forward, and when the thalamus stops, now there's the hemispheres with the septum in between. The septal area-- in humans it's very thin, but in rats and other rodents it's very thick. This is basal forebrain at the bottom. The olfactory tubercle, that's the ventral-most part of the striata, the part that's been there since the most ancient chordates. Getting the olfactory input and that's how olfaction originally and still influences behavior, by projecting into this region.
But notice other things in that region. If you go back a little bit you're in amygdala. And this node here, that the whole ventral medial striatum is, I've got it called here the same way as the basal forebrain because, in fact, they are all part of the ventral striatum. Usually we don't say the septum's part of this striatum because of it's embryonic origins, but the rest of these structures are all part of ventral striatum.
And if we go forward, where now it divides into two hemispheres, you can follow it. This is still our basal forebrain, that is the septum, and includes the most ventral medial part of it of the striatum here. Most of this we call ventral striatum. And it has specific structures in it, like nucleus accumbens, bed nucleus of the stria terminalis, the basal nucleus of Meynert, and so forth.
All right. It's nice to review these a little bit. I've not named everything. Let's talk about a couple of other things here quickly that you should understand a little bit. I'm interested in ways the hypothalamus can influence the neocortex. And I want you to remember the major reward pathway in mammalian CNS.
Can someone answer that? What's the major reward pathway, if you hear that phrase? We've known about it for many years. These are the axons that use dopamine as a transmitter. Where they coming from?
Here, when you talk about the ones coming from the ventral tegmental area here, OK. But in fact, the function of the ones that come from the nigra here-- the medial part of the nigra here perhaps should be shaded in pink here too because it's really part of the limbic system as well. They're really also a reward pathway, but of a different nature. Usually we're talking about the dopamine axons that go through the medial forebrain bundle in the lateral hypothalamus here, and project into the basal forebrain.
So what about this first one? Describe two ways the hypothalamus can influence the neocortex. Well actually, there's a lot of ways. You could say, well, the hypothalamus is connected in a two-directional way with limbic structures, subcortical limbic structures. And those are connected with, you could say, cortical and limbic structures including hippocampus, which are connected to the paralimbic areas, which are connected to the neocortical areas.
This is [INAUDIBLE] diagram, it shows just major routes from sensory areas. This is primary sensory areas here, the hypothalamus down here. First of all, we do expect the hypothalamus to influence neocortical area. And what kind of influence are we talking about?
Well, you know what functions the hypothalamus is involved in, so we're talking about influences of the mood, motivational states, and neocortical processes. And we know that moods, and especially motivational states, influence the way decisions are being made by neocortical systems. We may be talking about cognitive functions but you don't get away from motivation. You don't behave without motivation, and motivation often involves changes in emotional states as well.
All right. So here I outlined many different ways the hypothalamus can influence the neocortex. We just talked about Papez circuit. The more direct and the more indirect way through Papez circuit to get from hypothalamus to neocortex. Earlier we talked about the gating of information by hypothalamus, the gating of information going through the thalamus. These are the chapters where I mention that.
That's certainly a way the hypothalamus is influencing neocortex. We talked in chapter 17 about the widespread external systems. I mention a few of them here again. That's certainly an influence of hypothalamus from neocortex.
But then there's a very direct way, by way of the medial dorsal nucleus, and I show it here. Here's your hypothalamus down here. Here's the thalamus, and I'm showing here the medial dorsal nucleus which begets prefrontal areas of the cortex. And the medial part, which contains the larger cells, receives axons-- I've just shown them here on the left with a red arrow-- the hypothalamus projects as does olfactory system to that part of the medial dorsal nucleus.
So this is probably the most direct way hypothalamus can influence the neocortex. But all those inmost direct ways are very important as well. The influence is very widespread. OK.
So I point out here that a person's mental state can influence the endocrine organs by way of the hypothalamus. You've got to consider what we mean by mental state. Let's talk about how the hypothalamus can influence that mental state. The influences go in both directions. So that's one way they get experimental data on it is to look at disturbances of the hypothalamus during neurosurgical procedures, because often during neurosurgical procedures, the patient is conscious.
The brain doesn't contain pain receptors and pain pathways don't originate in the brain itself, so you can operate on the brain as long you anesthetize the structures that you have to open up to get in. OK, so you can get pretty drastic changes in autonomic activity, you can get changes in mood, changes in emotional expression, changes in feelings being reported. The founder of our department, [INAUDIBLE] always in his introductory lectures-- in 900-- would talk about a soldier during World War II who ended up with a piece of shrapnel in his third ventricle. Very difficult, at that time anyway, to get it out, so they left it there.
Every time he moved his head a lot, that piece of shrapnel would ride around inside his third ventricle and stimulate structures mechanically near the walls of the ventricle. And he would start crying uncontrollably. And it's only when he sat still for a while that that stimulation stopped. What interested me the most is that when you asked him why he was crying, he would say things like, well, it cries in me. In other words, that's not me crying, it's just something happening to me.
But later as time went on, he began identifying with his states and he would say, I feel really sad and unhappy and that's why I'm crying. And I think that's a real lesson. When things happen to us you don't really have to identify with the states, you can separate yourself from them. And that is the way you have more self-control.
Nauta goes on and he talks about the concepts of autonomic and voluntary. We talk about the voluntary nervous system for the primal tract, for example. It's part of the voluntary nervous system. We talk about the involuntary nervous system, the autonomic nervous system, and other subcortical or non extrapyramidal motor system, like involving cerebellum and striatum, and various structures of the brainstem.
And Nauta pointed out that that's a little bit misleading because, first of all, the so called voluntary nervous system-- corticospinal projections and so forth-- are engaged in unconscious habits, performance of habits that you can do with very little awareness. It's not just voluntary movements. I'm just pointing out that we don't fully understand what we mean when we talk about voluntary and involuntary in terms of brain structures. Similar to autonomic, it's usually things happening automatically, but in fact, some people get quite a bit of control out of their autonomic nervous system.
The best known is reported yogic exercises where people learn to control their heart rate, they can even change their body temperature. There's even some yogis that are able to make one hand warmer than the other, and things like that. But I point out here that it's very limited because most people can't do those things at all, at least not directly.
Nauta also points out that hypothalamus has very few long pathways either leading out of it or coming into it. I mentioned the long pathway carrying pain information, but that's very unusual. Most of them are polysynaptic unlike pyramidal tract, unlike the dorsal column medial lemniscus system which are long accents. Characteristic of hypothalamic connections are the shorter pathways.
One more topic here that you should know about. We talked about disconnecting hypothalamus from other structures. It was a very interesting experiment I did not talk about when we talked about those earlier experiments. That's particularly interesting to me because I'm interested in mechanisms for recovery. And I talked about diaschisis effects, well this involves that.
If you take a rabbit, and you can loop a wire-- it's a difficult surgical procedure, but you can get a wire in there that loops below the caudal end of the hypothalamus. So if the wire is pulled-- so now that animal's conscious, but he's got that wire-- so you can pull the wire and you're not causing any pain, but you're basically slicing the pathways between the hypothalamus and midbrain. And if you pull it up high enough, you'll completely disconnect the hypothalamus from the midbrain.
OK, if you do that in one quick step, you will have a lot of trouble keeping that animal alive because he can't directly control his feeding, his temperature, and various things like that that the hypothalamus is so important for. But if you do it in small steps, and wait every time you do a little more damage, you wait-- he will recover. And if you keep doing it, you'll eventually completely separate the hypothalamus from the midbrain, and he still recovers. That's called a multi-stage lesion.
What kind of damage to the brain can you think of that's like that in patients? Think of a tumor that's slow growing. One reason they're so difficult to detect and one reason a person will come to see the doctor only when it's huge is because it's like that wire being pulled really slowly he's recovering all the time from the damage being done.
[INAUDIBLE]?
That's one reason why people with Alzheimer's or people with Parkinson's, say, you don't notice the symptoms until the damage is very far along because it's happening gradually. It's a good parallel. All right. So that's Rudolph Powell was the one who did that with rabbits, that experiment I was just describing.
I just point out some of the things you should review, and I also showed the figure from a paper that studied how the central gray area of the midbrain, especially in an animal that's got this kind of disconnection, actually has different areas that serve, say, fight and flight, sexual behavior, defensive behavior, and even feeding. So it is possible for animals to recover a lot. The problem is if the midbrain-- they don't have much control of the endbrain at all, so they're completely abnormal and they will not initiate very much behavior, as we said before.
So that's one of my favorite quotes from Rumi, when we're studying hypothalamus, this is the way I feel. OK, so I will post the slides here that discuss hormonal and other influences. We'll spend just a little time at the beginning of the next class going over just a few things here. I think it's a very interesting topic and I know all of you are interested in it.
But read chapter 27, and then we'll go to chapter 28 because I need at least a full class to talk about hippocampus, so we'll do that in the second part of the next class. And then that's Friday, and then on Monday we'll finish talking about hippocampus, so we can talk about the amygdala and the basal forebrain.