Flash and JavaScript are required for this feature.
Download the track from iTunes U or the Internet Archive.
Description: This lecture is the fifth of five on the hypothalamus and limbic system and focuses on the amygdala and other aspects of the limbic forebrain.
Instructor: Gerard E. Schneider
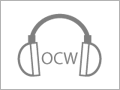
Lec 31: Hypothalamus and li...
The following content is provided under a Creative Commons license. Your support will help MIT OpenCourseWare continue to offer high quality educational resources for free. To make a donation or view additional materials from hundreds of MIT courses, visit MIT OpenCourseWare at ocw.mit.edu.
PROFESSOR: OK. Today we want to focus just on the hippocampal formation. These are a couple terms I want to make sure you're understanding the way I've been using them. Whenever we talk about the limbic system, we're talking about structures closely connected to the hypothalamus, so similarly in the endbrain. The limbic telencephalon always means the endbrain structure is strongly connected to the hypothalamus. It doesn't mean they're not interconnected. They usually are. But what they have most in common is their connections with the hypothalamus.
And when we use the term pallium it always means-- the word means a cloak or mantle, so similar to the meaning of cortex means bark. The bark of a tree is a cortex. So all the cortical structures of the endbrain are included. And the medial pallium includes the hippocampus. The medial pallium of the embryo, it's still called medial pallium in many non mammalian animals.
When we talk about basal forebrain-- and we'll talk more about that when we talk about amygdala too-- we don't talk about-- we don't call it the pallium. Even at the base there were the olfactory tubercle is somewhat cortical-like, we just call it the basal forebrain of parts of the ventral striatum. You'll see that in the way I label the pictures.
And these are the functional topics we'll be paying attention to. Spatial memory, place cells and head direction cells. We'll talk a little bit about synaptic change, especially synaptic enhancement and information storage in the hippocampus. And a little bit about some of the neurotransmitters involved, especially acetylcholine and the way they changed, for example, in sleep compared to waking.
All right. So first of all, you need to know differences between place cells and head direction cells. How are such cells defined? Well, they're defined by electrophysiologists. And this is a point, as I did for the auditory system also, if I wanted to talk about function I had to refer to physiological studies as well as anatomical studies. In fact, I can't make sense of the anatomical pathways without that physiological information, as well as some information from ablation studies of behavior.
So we knew about place cells long before we knew about head direction cells. But it was only when work on head direction cells began to appear-- less than two decades ago-- that we began understand more about these pathways. So we'll learn about the pathways whereby the shifts in head direction are signaled to the endbrain and hippocampus and why that's important.
And when we talk about place cells, I will try to remember-- I'm not sure if I answered these questions in the slides, but I'll try to remember to do it, but it is in the book-- the differences between dorsal and ventral hippocampuses. It's quite important. Basic differences that, in the more ventral hippocampus-- which in humans is the more anterior hippocampus because of the shift of hippocampus into the temporal lobe-- the connections to the amygdala are strong. Not true for the dorsal hippocampus. And the place cells represent larger places than in the dorsal hippocampus.
So this is an illustration of physiological-- from a physiological study of place cells where they record from a number of different neurons in the hippocampal formation. Usually they're recording from dorsal hippocampus because, when we say dorsal and ventral we're usually talking about a rat or a mouse or a hamster-- usually it's rats and mice that are used in these studies-- each of these little squares represents a room where the rat can run around. And he can see overhead, he can see where he is with regard to other things in that room. But remember, that's a whole room. OK?
Now, Matt is often using a runway and recording place cells represent places the rat can be on the runway. But here, when the rat moves around the room, you can see that many of these cells are only active-- when they're very active he covers the area in red. OK? If it's dark blue it means it's not active at all.
So these are place cells. Cells that are active when the animal is in one particular place. That means not where he is looking but where the animal is located. That's what a place cell is. And you can see some of them are very broadly tuned, some of them are very precisely tuned, represent a tiny little area. They tend to find these very dorsally in the hippocampus. That is at the septal end of the hippocampus, the one closest to the septum.
And there's some cells that they don't know what they're doing because they're not responding to place. OK? Some of them may be infected direction cells.
OK. So when we deal with head direction cells in the system, this is how we define it. Here's a rat and here we look at how his body accesses a line. That's this line. And the angle here theta is the angle of the animal from some landmark that the rat is using to orient himself. We do the same thing.
Even when you're not aware of the landmark you're using, we always do this. So we have a sense of where we are with respect to things in the environments. Usually sensed by the visual system. And there are specific structures in the-- not in the hippocampus itself, and usually not in visual cortex itself. There's not many projections from primary visual cortex into the system but there are from other areas that represent landmarks.
So theta is angle-- we call it allocentric direction. Not the direction where something is located with respect to the head. That's egocentric direction. This is allocentric direction. And now, if he turns his head by this amount delta theta, then this allocentric direction can be corrected if the animal-- now, what's of interest here is, what places is he headed towards with respect to the landmark? So this, then, is the corrected allocentric direction. It changes all the time depending on how his head is turned.
OK. So these are the things you need to know to understand what's going on. Now, here's the figure we saw before of the Papez circuit. Here is the allohippocampal formation, the part that Papez was familiar with would all be included here in the dashed line-- hippocampus itself, subiculum next to it, and then the dentate gyrus at the far end.
OK. Now, changes in head direction-- and this we talked about-- they're signaled by vestibular and probably [? proprioseptum ?] systems in the brain stem. They come in by way of the-- the so-called tegmental nuclei-- they're named after-- Gudden named them so they are often called the tegmental nuclei of Gudden-- in the column of the brain and they go through the mammillary bodies. And in the diagram, that's this pathway. Here's the tegmental nuclei, here's the connection to mammillary body.
So what's the mammillary body doing? Well, the information to the mammillary body is coming from hippocampus here. That is constantly giving the mammillary body information about theta. So the information from the tegmental nuclei then is-- changes that activity to theta plus delta theta. And that's the information on head direction signalled, that goes up to the cingulate cortex through the anterior nuclei of the thalamus. This is the [? mammillary ?] tract. OK?
So this is just what I said. The relevance, of course, is that the place-- the animal, in his internal map, the environment-- he is anticipating changes in position by those changes in head direction. So basically, what I think is happening, is that every time you turn your head, or an animal turns its head, you're aware of the places in that direction. And those places are associated with memories. So you're constantly-- that is happening through these connections, through the hippocampus, through these paralimbic areas, through the neocortex.
And I point out here that we've only characterized the head direction cells. There are other axons in those pathways that we don't fully understand. And it also raises the question, what's the hippocampus sending to other parts of the hypothalamus? I'd point out here that connections going to the septal area, to other parts of the hypothalamus, in addition to this larger connection to the mammillary body. So we know the major input to the mammillary bodies concerns allocentric head direction. OK?
It may alter motivational states according to the memories being activated about those potential places. And let's go back and talk about-- here we're talking about place memories. But early on in the book I talked about two major links between olfactory inputs and motor outputs. Both of which were classed-- they represented different kinds of learning. Two major links between-- this was before the evolution of visual and other inputs into the endbrain.
So through the ventral endbrain, which has become the corpus striatum and basal forebrain, that was one route. And we know that that's responsible for habits, and early on they were olfactory habits, responses to different olfactory cues. But there were also inputs to the pallium. The main part of the pallium was the medial pallium of the dorsal endbrain. In fact, the rest of it, what we call dorsal cortex in primitive animals, is really equivalent to parahippocampal regions in mammals. And the major output of those areas is to the medial pallium.
The medial pallium was really the dominant pallial structure dorsally. And even in advanced mammalian brains, if you look at gene expression studies, there are genes that are expressed in both the entire neocortex and medial pallium. And that medial pallium, of course, became the hippocampal formation. And we know that that is concerned with a very different kind of memory. Not the habits that the stratum is involved with, but memory for places initially in evolution.
This just takes that same outline and amplifies it a little bit. That medial pallium projects through the end of the ventral striatum, but also direct to the hypothalamus, also the epithalamus. Those links were [INAUDIBLE] two of the habits were different concerning disassociated place with good or bad consequences approaching those places.
When I talked about these major evolutionary steps, I mentioned early expansion of the endbrain, both striatal and pallial. And I showed two main pathways going caudally from the olfactory system. One of them goes to the base of the brain here. That would be equivalent in modern mammals to the pathway to the olfactory tubercle which is the very ventral most part of the ventral striatum.
And the other one goes up to the pallium. And that would be equivalent to olfactory projections to hippocampal formation. They exist even in mammals. Enhancers, I've traced pathways from the olfactory bulb to the very anterior most part of the hippocampus. It's called the hippocampal rudiment. That actually joins with the septum and the olfactory bulb projects directly to it.
So you can find remnants of these early pathways. We already talked about olfactory projections and I talked about how the olfactory bulb dominated the whole pallium in the-- say, in hagfish and the sea lamprey.
All right. Let's look at that medial pallium in these animals. The shark, the lungfish, the bullfrog and marsupial. There's only one mammal. I picked the marsupial here because he doesn't have a corpus callosum so he's most similar to these other animals in the way the cortex is arranged. OK?
And just look at the major change in the configuration of the medial pallium. In those animals-- the shark, lungfish and bullfrog-- we call the more primitive vertebrates, and then the hippocampus in mammals. We could say birds as well, but things have evolved quite differently in the endbrians, as you know, in birds so I'll be focusing on mammals more.
But here it is. I've colored it in pink from the studies that I was using. And here you see the dominance in size of the medial pallium compared with the rest of the dorsal cortex. This is olfactory cortex down here. That's lateral pallium. That's olfactory cortex. This is a striatal structure here and this is the septal area.
So there's the medial pallium of a shark. It's a spiny dogfish. It's a very small shark. And here in an African lungfish, again, if you look at the-- well this is actually olfactory bulb here-- but if you look at this level when you're behind the olfactory bulb, here you have the dorsal pallium. This is olfactory cortex, the lateral pallium, this is striatal. And there's the medial pallium. You'll see it here. It's thicker, it's more differentiated than the rest.
And here's a bullfrog. It's even more striking how thick the medial pallium is here compared to the rest of the non olfactory pallium. And by the way, on the bullfrog, that medial pallium does get some direct olfactory inputs. But much of it does not. The same is true of this dorsal cortex. It gets a little olfactory input directly out here.
Now, here is the Virginia opossum. So now we're talking about mammals, a mammal without any corpus callosum You would think he's a natural split brain animal, but in fact he isn't at all. Just the fact that he doesn't have a corpus callosum doesn't mean a whole lot because the axons still go from one hemisphere to another. How do they get there? Do any of you know?
If you're looking at, say, areas next to the primary visual areas, and even the striate cortex has some axons that go across the callosum, the ones representing the vertical meridian. But how do they get there in the opossum? How do they cross to the other side?
They go all the way forward into the anterior commissure, the structure in the basal forebrain. Ventral to most of the septal area. That's where they cross. So the anterior commissure is huge. All of these areas, this is all commissure axons here. It's so huge. It's not that big in any of the non mammalian-- in any of the mammalian animals except the various marsupials.
So here's the neocortex up here. You follow it around through a cortical area with fewer layers, the cingulate cortex. It's also got that very prominent layer two. And then, right here, you're suddenly through some transitional areas here. You're in hippocampal formation. And if you've followed the hippocampus around and cross-sectioned you come to the dentate gyrus. And the dentate gyrus, here at this level, abuts the septum. This is the septal area.
The only way you see that in the labs we-- the animals we study in the lab, the mouse and the rat primarily, is to look at the hippocampal rudiment which you find just above the corpus callosum. But around the front of the callosum, you'll see that structure coming down and joining with the septum the same way. This is much clearer in the marsupial where we can see it much further anteriorly also.
The hippocampus does get a lot bigger in this animal people if you go caudally. Because it's become much larger posteriorly where it's neat the visual areas. I already pointed out how important visual landmarks are to the function of that major function of the hippocampal formation in responding differently in different places. The importance of visual information has led to most of the expansion of the hippocampus next to the visual areas.
So here it is in a rat where you see the-- here they cut out cortex in this drawing to show the hippocampus underneath. And they're showing that if you make a cut across the longitudinal axis of the hippocampus-- you see there-- you'll always get a slice that contains the same circuit. And we're going to go through that circuit in more detail in a minute.
So if you look at it dorsally, if you want to get at the hippocampus, you have to cut a window through the cortex-- through the neocortex, and you expose the hippocampus underneath. You don't just go through and exposed cells. You have to go through the cortex. And this is just a drawing of that. They've blown it up.
OK. And here, this is a reconstruction of the hamster that I did. And here you see a dorsal view of the hemisphere. And there's where the hippocampus is. And then in orange I showed the axons coming from hippocampus going into the fornix. This is the columns of the fornix where they then come down, go through the caudal end of the septal area on their way to the mammillary bodies in the hypothalamus. And they also show axons crossing from one hippocampus to the other. The hippocampal commissure.
So here in the side view, there's the fat cigar of the hippocampus. Of fat banana, if you will. Ventral hippocampus, dorsal hippocampus, here are the fornix fibers coming out of the hippocampus, mostly from the subiculum, which we'll see the way it looks in the cell stain. It's really part of the hippocampus. And then there's the bend. This is going around the internal capsule. The internal capsule fibers that connect thalamus to the cortex are coming through here. There it goes-- it's forming this column, compact column, of fibers that you can follow all the way to the mammillary bodies there at the caudal end of the hypothalamus.
So this, of course, is underneath. This is, in the hemisphere, this is in the branch down here. And this just shows you someone's imagination more than an actual reconstruction of where that is in the rat brain.
And here they call this the-- this is the temporal end. You could call it the amygdalar end because it's the amygdala sits right there. OK? That's here and this is the temporal end in the hamster. And this is, they call it the septal end. Closest to the septum. Because the septum, you see, is right there. This is all septum.
There's the septal area here. And I show some of the axons that are leaving the rest of the-- we call this the precommissural fornix, the axons that leave the main bundle of the fornix columns and connect mainly with the septal area and other structures in the basal forebrain region.
So now we want to identify the major sub regions of the hippocampus and the adjacent structures, and look at the pattern of interconnectivity that everybody in the [INAUDIBLE] lab knows about because they study it and they look at changes in the various synapses. And these different regions, which you see here, are labeled CA1, CA2, CA3, and these loosely packed cells here, CA4. And then dentate gyrus.
Whenever you cut-- this is a horizontal section of a rat, and wherever you cut if you're cutting across the long axis-- meaning this way, this way, this way-- as long as you cut that way across the long axis, you're going to get sections that have this appearance. OK? So you see the same basic circuit at all these levels. And we'll follow that circuit.
But first, what does CA mean? Cornu Ammonis, the horn of Amon. Amon was a Greek god. I'm sorry, Egyptian god, sun god. And he carried a horn. So these names come from mythology. In this case, Egyptian mythology. So it means the horn of Amon.
Nowadays, students who do research on it sometimes don't even know who Amon was. Unless they read my book. They certainly know CA1, 2, 3 and 4 are, and what the dentate gyrus is.
But notice, if you start here at the dentate gyrus end, you go CA4 down to CA1 which ends right here. And then the configuration itself changes. This is called the subiculum, where the cells have this broader layer. It's really part of the hippocampus, but it's where the cells are broader. Well, most of the output into the fornix going to the mammillary bodies is actually coming from this area, from the subiculum.
And if you keep going, now you get additional layers. This is the presubiculum. This cortex. And then you get-- and they do name sometimes additional areas like parasubiculum, prosubiculum, you don't need to know all that. Subiculum, presubiculum, then you get to the entorhinal area. Entorhinal area is well known to physiologists who studied the system because it is the major bridge between neocortex-- association areas of neocortex. The major paralimbic area here is entorhinal. And that's the major route, especially this one through the dentate gyrus. But it also connects directly to the subiculum.
So if you look at question three here, I'm asking what's the name of the major afferent input pathway to the mammalian hippocampus? Where does it originate? That would be the pathway you should describe. OK? The one from entorhinal area that goes through this way into the dentate gyrus. It actually seems to pene-- this is actually a deep sulcus. But in evolution, axons have actually crossed directly there. So we call it the perforant path because it perforates what is actually peeled surface of the brain.
Let's look at that circuit. But before then, what's the difference in location of the hippocampus in large primates and it's location and it's location in the rat? Because I've been stressing here the rat. If you look at human, they've shown the hippocampus in red here-- the temporal end and this would be the septal end-- and notice that now, what is ventral in the rat is really anterior in the human because of this shift into the temporal lobe of the primarily visual areas.
Now, there's auditory cortex here too, but this is all visual cortex. It's the striated area here and then the areas next to striated-- B2, B3, B4-- and then you get into infratemporal areas all along here. This is all visual. The hippocampus can be followed all the way down here.
This equivalent to the ventral hippocampus, the anterior hippocampus in the primates, large primates, was the part nearest the amygdala there. And this is where the place cells are quite large. This is equivalent to more dorsal hippocampus. They show it as, I think, it's probably a little too narrow there because it's actually pretty substantial in humans. So that's where you get the smaller place cells.
And I said, what's the hippocampal rudiment? Here I've redone-- it's just to emphasize a little bit the hippocampal rudiment-- this is hippocampus continually all the way. At the very-- right above the [? callus, ?] all the way here to the septal nuclei, you can follow hippocampus. So you see the hippocampus is the medial pallium everywhere. For the entire-- everywhere in neocortex, if you follow it medially, you come to hippocampus. It's just that it's very small except when you're near the visual areas caudally. OK?
So we've answered number six here. And now I want to describe that circuit that begins in the entorhinal cortex and can be followed through the hippocampus to the subiculum. And from there, as I mentioned, the major output to the mammillary bodies starts. And then we want to answer this, where can physiologists get the changes in the synapse that we call on from potentiation? Just by rapidly simulating the pathway. Actually, they get it at every synapse through this pathway.
So here I've taken a picture drawn from Golgi material by Ramon y Cajal, and I'm picturing that pathway because I've colored in axons that he had numbered but he hadn't colored them, beginning with axon coming out of the white matter here. It's actually originated in the entorhinal cortex which is out here off to the right. So this is the first axon in the pathway coming from entorhinal cortex, here's this perforant pathway coming directly into the dentate gyrus where the axons then travel parallel to the surface after they enter and terminate on dendrites of the dentate gyrus.
Then I show a dentate gyrus cell here that's getting that input. And I'm showing it for this one. So here the input's coming into the dendrites, here's the axon of the dentate gyrus cell that you can follow, travels through the dendritic layer of the hippocampus, and it synapses on the CA3 neurons. This is CA3. CA4 is in this region, they hilus of the dentate gyrus. That's not where they terminate. They terminate on CA3 primarily.
And then, the cells in CA3 project-- through axon number three-- they project to cells in CA1. This cell here. And notice that that axon has a branch that goes right into the fornix. So areas other than the subiculum here do project into the fornix, including the CA3 cells.
So then here is-- so this would be the third axon in that pathway through the hippocampus and the CA3 cell to a CA1 cell which I show here. I'm not saying that it doesn't terminate on other cells here too. I'm showing one of them here to show an axon. And Cajal simply drew the output going into the fimbria of the fornix. In other words, through axons through the fornix.
But now we know that it also connects-- I drew it in purple because it wasn't in Cajal's original-- it goes through a subiculum cell which then has an axon going into the fimbria. And in fact, these cells provide the majority of those output axons that go to the mammillary bodies. But you see it's fairly complex, even in these simple Golgi drawings. And I had to draw this one in because it didn't stain as well in the Golgi stain that Cajal was using so he didn't see that pathway.
All right. Remember, I said that if you cut across the long axis, as you see there, you always see that pathway. And here they've drawn it again. Some of these drawings that you see in textbooks, they show these axons go directly in. Like they show this one going into the fornix. They show this one, similarly. But now we know even more of them come from the subiculum. And this is known from experimental work.
You sometimes miss some major things when you just use Golgi. You need to do experiments with labeled pathways and trace them. The imaging methods, too, live imaging would miss all that too. So we know this from experimental tracing studies.
So you can characterize the pathway, as I've shown at the bottom here. The dentate gyrus cells with their dendrites getting input from different parts of the-- through the perforant pathway, different parts of the entorhinal cortex. This is the lateral perforant pathway, the medial perforant pathway coming from different parts of the lateral and medial parts of the [? inferential ?] cortex.
They project then to dendrites in the CA3 region. And then this collateral, anyway, the Schaffer collateral. Named after, of course, a guy named Schaffer and his projecting to dendrites to CA1 which then projects to subiculum cells as you see here. And that shows an output and angular bundle going into the fornix and on to the mammillary bodies.
And I point out here that long-term potentiation can encourage at each of those synapses. This synapse, this synapse, this synapse, and this synapse. They've studied each connection separately in places like the Tonegawa Lab. They tend to focus on certain ones in their findings and their different functions and different components of the hippocampus. But a lot of that is still up in the air.
So we know about LTP, but if you think more broadly in terms of function, what we call behavioral studies call our memories, what kind of memory are we talking about? It's not the kind of habit memories that we talked about in striatum.
AUDIENCE: [INAUDIBLE]?
PROFESSOR: When we talk about long-term memory, yes. How is that different from-- I mean, I have a long-term memories learning how to manipulate a pencil too. Isn't that a long-term memory? Is that hippocampal?
AUDIENCE: It's not something like going out [INAUDIBLE]?
PROFESSOR: What kind?
AUDIENCE: [INAUDIBLE] memory.
PROFESSOR: It's a striatal memory. In terms of the anatomy, that's a striatal memory. Learning how to ride a bike, learning a sport, these actions where you don't remember a specific time and place, you remember a movement. So whenever you're learning any movement. I'm not saying that right at the beginning there aren't multiple types of memory involved. But someone with hippocampal lesions, someone like Henry M. who had major hippocampal damage, he couldn't perform normal long-term memories but you could teach him sensory motor habits.
OK? Simply reward him repeatedly. He wouldn't remember being in the learning situation, he wouldn't remember the teacher, but he would gain those skills. That's one of the types of research that's clearly separated these two kinds of memory. And it's the striatum that's the main brain areas that that happens.
Now, there's a pathway where the sub-cortical projections go to and from the hippocampus that is not hypothalamus. And it uses acetylcholine as a neurotransmitter. It's a neuromodulator-- a major neuromodulator in the hippocampus. It's this pathway from the septal area here back to the hippocampus. And I show that-- I show pathways going from the hippocampal formation to septum and I show this acetylcholine pathway going from septalary to hippocampus. That's the answer to this question.
And this is from on human. And I know these human things are a little more confusing but this is what you get in medical schools text so I show them once in a while. This is the septal area shown here, this is the arch-- the arch of fornication, remember? The fornix-- arch of the fornix fibers. And there, in purple, I've drawn acetylcholine axons that project right into the hippocampus. And then there are pathways going back directly to the septum [? pronate ?] from the hippocampus and from the subiculum.
And then this diagram also shows this major input from the entorhinal area. It's getting cortical inputs into the hippocampus. You get other kinds of plasticity. One of the most dramatic kinds of collateral sprouting that's been seen in the adult brain occurs in the hippocampus. And I can characterize it like this. Well, let's look at this one first.
Here are some cells in the dentate gyrus. And here's a box. Each of that box corresponds to these boxes. And here I show inputs coming from other parts of the hippocampus. These are associationaries.
Now, the entorhinal cortex, it projects up here. So what happens when if we remove all the entorhinal projections? You get collateral sprouting of these other axons coming down here. And so I show in red the sprouting that occurs. Major sprouting. It's a huge change in the projection.
So if we look here, here's the terminations of-- this is that imagine the dentate gyrus cell here and here it's dendrites in every one of these boxes. So here's all the input from entorhinal cortex. And here's where the association axons terminate. So we get rid of all of these. That's what's shown here. All of these are eliminated.
Here's what happens to the association axons. And that's what I'm showing here. Huge amount of sprouting. And it's not just the associationary area's axons that sprout. Other systems also sprout. So it's very dramatic adult plasticity.
And now, people that have studied plasticity after-- in physiological situations, changes in synapse are beginning to find anatomical changes also. So it's not only after these drastic lesions that you get anatomical change in the hippocampus.
And then you should go through this book. It goes through this amazing finding that we have a lot of memory consolidation. Consolidation of long-term memories for things that happened during the day are consolidated largely during the night. And I'm pointing out how the levels of these modulators changes. In waking, slow wave sleep, and rapid eye movement or dream sleep, here's the acetylcholine levels.
And rapid eye movement levels are quite high. Even higher than waking. But in slow wave sleep they're very low. And this is the period where you get that consolidation happening. And the reason that people that have woven these for periods trying to explain why this occurs. We know it occurs. We think it's because during slow wave sleep, there's a lot more [INAUDIBLE] information from hippocampal structures. These are the hippocampal structures back to cortex.
Showing you here. Whereas with high ACH levels, the same pathways are active but much less so with that modulator present. And a lot more-- the flow from neocortex to hippocampus is still strong. But the flow back to the cortex is stronger during slow wave sleep. Which could explain some of that memory consolidation.
Because a lot of the-- it is retained in the hippocampus itself initially. But the hippocampus doesn't have the capacity to retain all our long-term memories forever. It's stored in the neocortex. And we know that from studies of electrical stimulation of the human brain during neurosurgery, from studies of damage in neocortex. And this just shows you another way people have represented these pathways between entorhinal cortex and hippocampal formation.
So neocortex, the hippocampus is critical in the whole way this thing works. And we know that both theoretically and from actual anatomical studies of various mammals. And I've just given you various pictures here. You'll see some humans. Some of you will be interested in the way it looks in imaging. This just shows where the amygdala is there. And here you see both the amygdala-- the almond shape right here-- and hippocampus in a sagittal view in an MRI scan. The radiologist become very adept at finding all these structures even with the low resolution you get in these types of images.
But what we'll do next time. I'll just review at the beginning the hippocampal stuff but I don't want to spend a lot of time on it. I will post these slides early so we can study more the amygdala here and its importance in this whole system. And then the structures in the basal forebrain anteriorly that the amygdala's strongly connected to. OK? So I'll post all these online, but also post the next class's things just to make sure we can get through it during the class.