Flash and JavaScript are required for this feature.
Download the track from iTunes U or the Internet Archive.
Lecturer: Prof. Hazel Sive
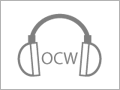
26: Nervous System 2
Related Resources
Biology terms (PDF)
We're going to talk about the nervous, and continue to talk about the nervous system. And in the game board of life and our journey through life we are well into the course. We've talked about the foundations, where things come from. And now we're talking about how different organs work together in the system module. We'll talk about the nervous system. Professor Jacks will move on into the immune system, probably not immediately after, but later into the immune system. And you're going to get a broader perspective on how things work in a biology sense. So we talked last time about the notion of the nervous system and how the nervous system is really a wiring diagram, a circuit. And it's actually, the circuitry in the nervous system, if you were to take wires and connectors and connect it up is very simple. What's not simple is that the circuit is incredibly complex. Enormous, of enormous complexity. And what's also not simple is that unlike many electrical circuits, it's a very flexible circuit. And, unlike most electrical circuits, it learns. It's plastic. It learns from its mistakes and it learns from its triumphs. And that's where the difference comes in. We talked last time about neurons as the wires of the nervous system. And today I want to talk about synapses, or you might hear them pronounced synapses, but this is an unusual pronunciation. Synapses, which are the connectors between the different wires. And then next time we'll talk about how these things are all put together into circuits. And that will be in our lecture on Friday. Actually, Claudette, did you want me to make an announcement about what you have on the board there? Can you guys see this? I can read it. It says RO6 Lesley's 10:00 AM, R16 Kyle's 11:00 AM. Go to 8119 tomorrow and next Thursday. OK? So if you are in Kyle's or Lesley's section, please note what is on the board. OK. So what about the number of connections? So this is a staggering figure. So the figure of the number of cells in our body ranges between ten to the twelfth and ten to the thirteenth. The number of neurons that are estimated to be in the brain is about ten to the tenth. Each neuron can make about ten to the third, a thousand synapses. But in some neurons there can be even more than that. There can be about, there can be up to about ten to the fifth connections between neurons. And if you start doing some simple math you can get to a realization that the complexity of the circuitry that you can get between neurons, just within the brain, is really staggering. OK? And it's way beyond our understanding right now to figure out how you get, at least these ten to the third connections, ten to the thirteenth connections. But wait. It may be a couple of orders of magnitude more than that, how you get those set up and how you get them maintained. So let's try to simplify the problem a bit and talk about the connections between neurons because that is a much simpler question than how you get this enormous number of circuits set up as you wire the nervous system. This is a simple circuit that I took from your book. A diagram of a simple circuit that I took from your book, motor neurons that are in the spinal cord innervate the leg muscle. Sensory neurons that are in the leg muscle innervate the spinal cord, and there is connectivity between the muscle, this sensory neuron and the motor neurons in the spinal cord that give you the classic reflex when the doctor taps your knee. I don't even know. I guess they do that when you're little, not when you're older, unless you look like you're in bad shape. But that is a simple reflex arch that has a number of connections between neurons but accountable number of connections, just a few kinds of synapses. So let's talk about the synapse. And I'm going to do some board work here and give you a sense of what the wiring in the nervous system looks like and where these connections take place. And one needs to consider some kind of input which can be from a neuron or it can be from some kind of external stimulus like light or the food you eat. And this input interfaces with sensory neurons, hence the name sensing. Sensory neurons generally connect to things called interneurons which are wires that connect one part of the nervous system to another. These connect to motor neurons. And motor neurons are the things that direct what the output is going to be, muscle contraction, swallowing, etc. Wherever there is a connection between neurons there is a synapse. And often, especially in the case of muscle, there can be a synapse between motor neurons and their output. There are two kinds of connections between neurons, two kinds of synapses. There are those that are electrical where the electrical input from the axon of one neuron is transferred to the next neuron, and you keep the signal an electric one. Electrical synapses are very rapid. They involve things called GAP junctions, which we've talked about briefly, which are direct connections between cells. And you get direct movement of ions between cells. And electrical synapses are used at some points in the Animal Kingdom. And they are, in fact, used in our nervous system, particularly when it's developing. This is new work. But the type of synapse that is much more prevalent is the chemical synapse. Chemical synapses are relatively slow. So electrical synapses are extremely rapid and they are almost instantaneous. You get the action potential moving down an axon, moves across the synapse, and there is really no break in the sequence or in the timing of the movement of the signal. Chemical synapses are slower. And there is a lag of milliseconds to minutes, in some cases, from the receipt of the signal at the one side of the synapse to its transference to the other side of the synapse. So mini-seconds. That should say seconds, S-E-C, to minutes. And the thing about them, about chemical synapses that makes them so attractive is that you can regulate them in various ways. So I'll say regulatable. And I'm going to spend this lecture talking about chemical synapses and not about electrical synapses. If you're interested in knowing more about electrical synapses, email me and I'll direct you to some literature that will be of interest to you. So let me draw on the board the essence of a synapse. And let's draw, and you should draw it at the same time. And so let's draw the axon of one neuron. So this is neuron one and this is its axon. And here's another neuron. And this is going to be a part of the neuron termed [the 41? . I have a new item. I have a new item as an incentive. I have, since it's getting to be summer, I have a clownfish, a clownfish squirt. You will need a bottle of water to use this item. OK. What part of the next neuron is the axon going to connect to? Isn't it the dendrites? It is indeed the dendrite. Yes. Thank you. OK. OK. So here is neuron two and the dendrite. And what is going to happen is stuff that is really not specific to the nervous system, except in the details. It is stuff that you've heard about in cell biology, that we've talked about in the formation module, and it involves receptor ligand interactions and signal transduction but in a specialized way. Here comes the action potential, hereafter abbreviated AP, coming down neuron one. And that action potential, as you remember, is driven by transient depolarization and inward sodium current. At the very terminal of this axon, which is called the presynaptic terminal or the presynaptic cell, there is a system of vesicles. And these vesicles contain stuff called neurotransmitter, which we'll talk more about in a moment. And on the opposite cell, which has the name of the postsynaptic cell, on its membrane are things that we've talked about many times, receptors. And this is the deal. The action potential moves along the axon of the presynaptic cell. As it gets towards the terminus of the presynaptic cell, you can call that the presynaptic terminus also, it activates another set of channels. These are calcium channels that give an inward calcium flow, an inward calcium ion flux that causes these vesicles to fuse with the presynaptic membrane in the process that you learned about in cell biology called exocytosis. And these presynaptic vesicles disgorge their contents, their neurotransmitter into the space between the presynaptic cell and the postsynaptic cell. This space is called the synaptic cleft or the presynaptic cleft. It doesn't matter. Well, it does matter. You should know that, but it's just a space. And it's a space that is usually about ten to twenty nanometers. So it's a fairly substantial space. And that neurotransmitter diffuses across the space, binds to receptors and signal transduction happens. And we'll talk about what the signal transduction is and what the outcome may be. And the outcome may be an action potential, but it is not guaranteed to be an action potential. And the thing about chemical synapses that makes them slow is that there is a diffusion driven process. The stuff has to, these neurotransmitter molecules have to diffuse across the synaptic cleft, and that takes time. And that is why chemical synapses is slow. You also have to get exocytosis of those vesicles, and that is slow as well. All right. So synaptic density. Here's a slide to show you synaptic density. Increase in synaptic density during development is really extraordinary. And the number of synapses and the type of synapses changes in childhood but also throughout life. This is a movie to illustrate what I've told you. You can look at it again. And graphically here's your electrical message being transferred into a chemical message, and then recreating an electrical message. So there's change in the transduction mechanism of this particular, oh, it's supposed to be a loop but I guess it's not, in this particular mechanism of transmission. Here are the presynaptic vesicles disgorging their neurotransmitter. Look in this case, the neurotransmitter is going back into the vesicles. And we'll talk about this more in a moment. OK. Item one on your handout today is a diagram from your book, which shows you the synapse, the presynaptic cell, the postsynaptic cell. You'll get all the information that I have put on the board in a more complicated way. And this is done for a cell that has got something called acetyl-CoA in it. OK. So you can bear that in mind. I want to address a number of issues now, a number of issues now. And I want to point out a diagram, actually, let me go back to this one, number one on your handout. One thing I would like you to look at and to think about and to compare with your cell biology module is the exocytosis of these vesicles. So you talked about transport of molecules around the cell, particularly proteins, and you talked some about exocytosis and endocytosis. The exocytosis of these neurotransmitter vesicles is a classic example of exocytosis. The proteins involved in this are the same proteins involved in exocytosis in many different cell types. In addition, there's a process I'll mention later on of endocytosis where the neurotransmitter may be taken back up into the cell and used again. On your handout that's on the Web is this diagram. You can look at it in more detail. I'm not going to dwell on it here. You can look at it in more detail. It will indicate where calcium comes into the triggered exocytosis of the neuro transmitter. But moving right on, I want to talk to you now about neurotransmitters. Neurotransmitters, the ligands that transfer a signal from one neuron to another. Here are some facts. Any kind of neuron can make more than one neurotransmitter. And different neurotransmitters are usually in different vesicles. And they can be used at different times and different places in the neuron where it synapses onto another neuron. Neurotransmitters are typically three things, and you'll be surprised at what some of them are. It's very interesting. They can be nucleosides, they can be amino acids, and they can be peptides. Let that on the table here. All right. So here are some neurotransmitters. Most of these, you can go and look at these later. Most of these come from amino acids. Acetylcholine which is one of the largest most important neurotransmitters does not, but the other ones do. They come from glycine from glutamate, tyrosine, tryptophan, and so on. And that monosodium glutamate that you have often on food, on Asian food that makes the food taste better, the reason it makes the food taste better is that it's a neurotransmitter and it increases synaptic transmission across some of the neurons that are involved and taste perception. So, yeah. All right, so neurotransmitters. Peptides, amino acids and nucleosides. It's very interesting that these ligands are very small. Amino acids are very small. Nucleosides are very small. And it's interesting that these are used as neurotransmitters. And I think there are two reasons. One of the reasons is that because they're small they diffuse relatively rapidly, and so they minimize the time of getting the synapse in the postsynaptic cell activated. And also because nerve cells are evolutionarily very ancient. These were the molecules that were around at the time, and perhaps they were around at a time where it was easiest to use things that were preexisting. So in some ancestral cell, which we don't really understand, perhaps before there was a very large protein cohort and cells wanted to communicate with one another, a very ancestral type cell. These were the types of things that would have been around. And these, perhaps there's an evolutionary reason that these things are used as neurotransmitters. There are a vast number of neurotransmitters. There are about 25 known neurotransmitters, and they have different functions. So the two big classes you should know about are the ones that are excitatory and the ones that are inhibitory. And we'll talk about this in detail in a moment, but excitatory neurotransmitters are ones which will promote an action potential in the postsynaptic cell. And, in fact, the major one in the central nervous system is glutamate. And the major one that works between your nerve cells and your muscles, at the neuromuscular junction, is acetylcholine. And then there are inhibitory neurotransmitters, and these are the ones which will tend to inhibit an action potential from taking place in the postsynaptic cell. And one of the major ones there is gamma-aminobutyric acid, abbreviated GABA. And another one is glycine. And those are both found in the central nervous system. However, I'm going to also add to this inhibitory list acetylcholine, which will indicate an important principle you should understand. And you'll see it gets more and more complicated, that neurotransmitters can either be excitatory or they can be inhibitory depending on the receptors to which they bind. This is a table from your book. You should look at it. You do not need to memorize what each of these neurotransmitters does, but you should become familiar with their names. And you will be interested to read what some of them do. And I'll come back to a couple of them later. I'll come back to acetylcholine, already mentioned. Serotonin which is involved in promoting good mood. And I'll also talk about adenosine as something that you affect every morning when you drink a cup of coffee. But before we get there, let's talk about receptors. And let me introduce you to two classes of receptors that you should be familiar with. So you could imagine, as we've been talking about, that the receptors for these neurotransmitters could directly influence an action potential in the next cell by allowing ions in or out of the cell. And, in fact, I'm going to add it to your first diagram. A lot of the receptors have eventually the effect of allowing sodium to flow in. But these receptors also may allow the influx of calcium or they may allow the efflux, the removal of chloride. And we'll talk more about this and I've got diagrams for you in a moment. So the receptors in general have got something to do with ion channels. And they can either be ion channels themselves directly. And in this case they get the name ionotropic receptors or they can be indirect and they can influence the activity of ion channels in an indirect way. And in this case they are called metabotropic. Metabotropic. OK. So the end result is that ion channel activity is changed. Ionotropic receptors, because they are the ion channels respond to neurotransmitters very rapidly. Within the millisecond timeframe, metabotropic receptors are much slower and they respond much more slowly within the second to minute range. And the deal with metabotropic receptors, I'll talk about both of them with pictures in a moment. The deal with metabotropic receptors is that between the receptor ligand interaction and activation of the ion channel, so the ligand will bind a metabotropic receptor. And the metabotropic receptor will then very often activate the system of G-proteins that you've talked about previously. And those G-proteins will then activate downstream ion channels. Here's an example of the acetylcholine receptor, which was the first receptor, neurotransmitter receptor to be purified. This is an ionotropic receptor. It's a complex of multiple subunits. And it works in a way that we talked about long ago when we talked about enzymes. And we talked about allosteric activation of enzymes. Remember that way back when? Something binds to a protein and changes its confirmation and changes the activity of the protein. That's exactly what happens here. Acetylcholine binds to the receptor in defined places, it changes the confirmation of various subunits, and going from a closed channel it now opens this channel. So this is a gated channel. OK? It's a gated channel in the same way that the sodium channel we talked about was gated, but it's gated not by voltage but by neurotransmitter binding. All right? That is the definition of gated. It can be gated by anything. So this is the acetylcholine receptor. It's also called the nicotinic receptor because it is the thing that binds nicotine, and can be activated by binding of nicotine. Metabotropic receptors activate ion channels. Here is something from your book. And I have these on your handout. So these are number two and three on your handout. Here is the neurotransmitter binding a receptor, interacting with various G-proteins, hydrolysis of GTP. One of the subunits goes off, and through a series of events opens up another ion channel that is not linked physically to the receptor. Metabotropic receptors. Now, interestingly, and here is another note you can make, a neurotransmitter can either activate an ionotropic or a metabotropic or both types of receptors. Acetylcholine is ionotropic. It can also be metabotropic. In hot muscle there are these things called muscarinic acetylcholine receptors, and they are responsible for slowing down or speeding up the contraction of the heart muscle. So there is a lesson there. Receptors can be either or both ionotropic or metabotropic. So up on the top board I've mentioned the terms excitatory and inhibitory. Let's go back to them and let's talk about excitatory versus inhibitory synapses. So the deal is this. You've got your signal coming along, you've got your next cell, or you've got your signal coming along, you've got your next cell here, you're passing the information to the next cell. And the question that that next cell is trying to answer is should I fire an action potential? That is the only question it is capable of answering. That is the only output that has real relevance for a cell, OK, is whether or not it's going to fire an action potential and transmit the nervous information along the pathway of the nervous system. And in order to decide that there are two things that can happen. Neurotransmitter receptor interaction can promote, can facilitate, can make easier an action potential to happen in the postsynaptic cell -- -- or can inhibit an action potential from occurring in the postsynaptic cell. So let's go back to our discussion from last week where we talked about resting potential, threshold potential and so on. And let me give you some information. The threshold potential is constant. For one fish, could somebody please define for me the threshold potential somewhere up here? Threshold potential. Yeah. Yes, you're correct. The threshold potential is around negative 50 millivolts where outside is more positive than inside. What is this threshold potential? What happens when you cross it? Yeah? And action potential is created. Right. Good. OK. So one first for you. And see me for another fish later on. You came up here with one. OK. The threshold potential, the point of no return at which the action potential is going to take place is constant from cell to cell. It doesn't change. What can change is the resting potential. And excitatory and inhibitory synapses act upon the resting potential and bring the resting potential closer or further away from the threshold potential. So excitatory synapses and the interaction of neurotransmitters with the receptors inhibit, OK, excitatory synapses will bring the resting potential closer to the threshold potential. They'll make it easier for an action potential to take place. So they increase the resting potential. RP for resting potential. Inhibitory synapses decrease the resting potential and make it harder to get to the threshold potential. OK? So you increase the resting potential and you still get closer to TP or the threshold potential. And you here you get further from the threshold potential. And the way you do this is by acting on specific channels directly or indirectly. If you're going to increase the resting potential so that you get closer to the threshold potential the channels involved are sodium and calcium. And those two ions will flow into the neuron. If you are going to decrease the resting potential, make it harder to get to that threshold potential, you want to do the opposite. So you could take sodium or calcium out, but that's not how the cell usually does it. The way the cell does it is to open up chloride channels. There's a lot of chloride. I'll show you some diagrams in a moment. There's a lot of chloride on the outside of the axon. It opens chloride channels and the chloride will flow into the cell. All right. Let's look at some pictures and some of the things I've drawn for you. So this is a diagram from last lecture which I drew for you. It shows you the action potential and it shows you the transient depolarization and reversal of charge distribution across the membrane. As a couple of you pointed out to me this is not accurate. Of course it's not accurate. It's a cartoon. And it's not accurate specifically because things aren't all or none. It's not entirely positive outside and entirely negative inside. And there are a number of different ions involved. So I have had a go at making it slightly more accurate. And this is number four on your handout. And now I've drawn it in terms of synapses. It's a complicated diagram but we can work through it. Outside the cell or in the synaptic cleft. And here's your postsynaptic cell. Again, this line as previously is the membrane. So here is your ion distribution. Outside the cell there is high sodium. Inside the cell low sodium shown in red. Inside the cell high potassium. Outside relatively low potassium. And you remember that that potassium-sodium gradient is constantly being generated by the sodium-potassium pump, and then potassium is flowing outwards according to its gradient through open potassium channels. That is going on all the time. I haven't indicated that, but that's going on all the time. In addition, outside the cell are high levels of calcium in brown and high levels of chloride ion shown in gray. Inside the cell there are negative charges. A lot of them are on proteins rather than free ions. OK. And I've shown you your resting potential is around minus 60 millivolts and your threshold potential around minus 50. So let's look what happens at an excitatory synapse. And the thing that you want to look for are channels that will hypopolarize, that will make the potential difference lower so that you get closer to that threshold potential. And the way you do that is to open up a series of gated sodium channels. Those same voltage gated sodium channels which we previously talked about. But I should tell you that there are dozens of different channels that are gated. And different cells have different types of gated channels but the idea is the same. These are voltage-gated sodium channels, and they are going to allow an inward flow of positive ions. In addition, there are gated calcium channels which will allow an inward flow of calcium. So this is an excitatory synapse. And you will get here a hypopolarization. Conversely, on your next handout and number six, at an inhibitory synapse channels that hypopolarize, that make you more negative will open. And these particularly are chloride channels that will give you an inward flow of chloride ion and make the inside of the postsynaptic cell even more negative and move it even further away from threshold potential. This is a diagram from your book I'm not going to dwell on. And I want to point out here again that I mentioned up here acetylcholine can either be excitatory or inhibitory. At the neuromuscular junction acetylcholine is an excitatory neurotransmitter. Here it is increasing or making less negative the membrane potential. So it is pushing you closer towards threshold. And here in the heart muscle it is acting as an inhibitory neurotransmitter making the membrane potential, the resting potential much lower. OK. So I want to move on now to something called summation. So we're building up the sense of whether a neuron, once it makes the synapse is going to fire an action potential. And that depends on this excitatory versus inhibitory stuff. But there's anther wrinkle, and the other wrinkle is the one that I alluded to and I've told you on the first board neurons make lots of synapses. They're not just making one synapse. They're making lots of synapses. And the input from many synapses needs to be added up, needs to be summated in order that the neuron decides whether it's going to fire or not. And that summation can be two-fold. It can be spatial in that a number of different neurons, a number of different synapses are made with the same neuron. And the sum of all the changes in the membrane potential are added up. And if you get above the threshold potential then you fire an action potential. Or they can be temporal where over a period of millisecond all the inputs into a neuron are added up. And if at the end of that time period you are above threshold potential then an action potential fires. This is number seven on your handout. Here is an axon. Actually, here is a neuron. Here are a bunch of synapses. They've said that they're excitatory synapses coming into the axon. The synapses can actually occur at many different places. In an axon they've shown them here coming into a part of the cell body. And there's this thing called the axon hillock which has got a particular configuration of channels. And that's the place where the cell often makes the decision as to whether it is going to fire an action potential or not. But it is misleading to see these synapses coming in all together because they can come in at multiple different places on the neuron. OK. So here are synapses coming in. And you sum up the input from these. This is the next diagram on your handout. So here in a graphic way are a number of small changes in membrane potential from a number of different synapses indicated by the numbers here. And these are summated at the axon hillock. And then the decision as to whether or not you're above threshold and whether you make an action potential is made. You can also do this over a period of time, again, on the millisecond time scale, and that will tell you whether an action potential fires or does not fire. OK. So the thing you need to remember is that the action potential is the output, and the decision is whether you make an action potential or not. So the decision to make an action potential. And the other thing that I should note is your action potential is your action potential. It has the same magnitude, and it's propagated once it starts. What you can change is the frequency with which you make that action potential. So you can have action potentials arising at slow rate or you can have them arising very quickly. So the decision is to make the action potential, it's all or none. I mentioned this last time. But you can change the frequency at which action potentials fire. All right. Controlling neurotransmitter activity. Neurotransmitters, here's acetylcholine and here is atropine, which is an inhibitor of acetylcholine receptor activity. Acetylcholine is an example of a neurotransmitter that is made and then destroyed. There it is being made, being used for receptor binding and for doing whatever it's going to do in the postsynaptic cell. And here is an enzyme called acetylcholinesterase that cleaves the acetylcholine and prevents it from being used again. Acetylcholinesterase is essential to acetylcholine function. Nerve gases, very typically, inhibit acetylcholinesterase. That is a very popular way that nerve gases work. And when they do so, what happens is that you have an excess of acetylcholine around and you get occupation of the acetylcholine receptors on the postsynaptic membrane that makes that postsynaptic cell fire repeatedly or fire without stopping. And that will inhibit your ability to breathe and not breathe and breathe and not breathe. And you will not be able to get contraction and relaxation and contraction and relaxation of the muscles that allow you to breathe. And nerve gases are generally toxic for that reason. They paralyze the muscles. Our troops in Iraq and elsewhere have in their pockets atropine syringes. Syringes filled with a substance called atropine. Atropine is a nerve gas antidote. And the way it works is in the following way. It acts by blocking. It's a competitive inhibitor of the acetylcholine receptor. And so even in the presence of nerve gas, the acetylcholine that is free and floating around in the synaptic cleft will not be able to do its thing because atropine is preventing it from doing so. Now, it's interesting. I've told you acetylcholine does many things through both ionotropic and metabotropic receptors. And atropine will affect all of these things. OK? It will affect the ionotropic receptors particularly, but what is has the side effect of doing is making you very sleepy. Nonetheless, it will save your life. OK. Serotonin, another neurotransmitter involved in mood. It makes you feel good about things. Here are two examples of ways that serotonin activity is regulated. Prozac is a class of molecules called a specific serotonin reuptake inhibitor. Serotonin, when it is made, is released and then is retaken up into the vesicles and used again. Prozac slows down this reuptake and allows the serotonin to act for a more prolonged period than it normally would have. And here is another one that you're very familiar with, caffeine. Caffeine is a competitive inhibitor of the neurotransmitter adenosine. Here is adenosine and here is caffeine, and you can see that they both have this purine ring. Caffeine will bind to the adenosine receptor. Adenosine normally promotes signal transduction pathways that make you sleepy. And adenosine can be counteracted by caffeine. You can go and look later at the pathway that caffeine and adenosine both act upon. And I will talk more about this next time.