Flash and JavaScript are required for this feature.
Download the track from iTunes U or the Internet Archive.
Lecturer: Prof. Hazel Sive
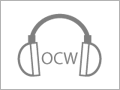
20: Fertilization/Fertility
Related Resources
Biology terms (PDF)
What I'd like to do today, is to give you an epilogue, the very end of the last lecture on cell type, that I did not want to cram into the very last one minute, so I'm going to take five minutes now to talk about that, and then I want to move on to a topic that is very related, because everything, of course, is very much related by the control of gene expression, by cell-cell interactions, by cell fate, and so on, but I want to talk to you about a particularly important set of interactions between cells. Here we are, on your game board of life, we're moving up through the formation module, talked about cell type, and we're going to build on that conversation in talking about fertilization today. So let me go back and remind you where we were. At the very end of last lecture we were talking about cell type, and what I wanted to do with you, was to trace for you the ontogeny, the development of a particular type of cell, and indicate to you how many different interactions there were between cells -- -- how many different sets of genes had to be activated, and had to interact with one another, to get you from a fertilized egg, all the way to a differentiated cell type, and I used the paradigm of skeletal muscle. And really, the point that I think you got, was that this is very complicated, and if you go on in your careers and think about, as engineers for example, as biologists, engineering a particular kind of cell from another kind of cell, you really have to know these steps along the way, and ask whether or not in order to get from point a to point b, you have to go through the whole set of 30 steps -- -- or whether you can circumvent those steps, and short-circuit, and go on a much shorter route. And we'll talk more about this in subsequent lectures, but this is very relevant for thinking about how to engineer particular cell types. What we were talking about was an extraordinary experiment that was done in the 1920's, that demonstrated a piece of embryonic tissue called the organizer of the dorsal mesoderm, was able to activate the formation of a second -- [PAUSE[ -- axis, or a second conjoined embryo, where most of the second embryo was derived from the host tissue, into which this piece of organizer, or dorsal mesoderm, had been transplanted. And this organizer was able to induce a second embryo, including skeletal muscle, must be made from the host cells. OK, who does not have their handouts here from the last lecture? I'm not trying to nail you, I just want to know if I need to write something on the board. I need to write something on the board, OK. So, the notion that we were left with was that, until now, we had gotten to the point where genetic information that had defined the dorsal part of the embryo, synergized with genetic information that formed the dorsal mesoderm that formed the mesoderm. That gave rise to this piece of tissue called dorsal mesoderm, abbreviated meso, which is also known as the organizer. And this piece of dorsal mesoderm, or organizer, itself, as I said, a very powerful inducing center, and it induces formation of a group of tissue blocks called somites. These somites, as I'll show you in a moment, are segments, or segmental units, that are present along the whole axis of the body, the whole trunk region of the body of vertebras. And they actually hark back to our ancestry, derived from organisms that were fully segmented, worms for example, earthworm type animals, that had multiple segments. OK. The somites, in turn, become subdivided, and I put this in just to indicate, again, the complexity of the process, and part of the somite, termed the myotome, activates the expression of the transcription factor myoD. And it was pointed out to me by Christine that I was abbreviating transcription factor, TXN that is my abbreviation, many of you may remember from way back, for transcription. So, if you have in your previous notes, a TXN, and you're not sure what I meant, I meant transcription. OK, so these somites get subdivided, they make the myotome that expresses myoD, and as we talked about last time, we come in full circle to where we wanted to be, which is the activation of the skeletal muscle program. Let me show you this on this PowerPoint slide. OK, so here are the organizers in diagram, activating the formation, or inducing the formation, of these somites. The somites get divided, myoD is expressed, myoD and other family members, remember I made the point this was a member of a redundant transcription factor, a redundant gene family, myoD maintains its own expression through a positive alter regulatory route. That means it binds to its own promoter, and perpetuates, or activates, its own transcription, so once myoD is expressed, it maintains its own expression, a very popular way of stabilizing gene expression in particular cell types. OK, here are some pictures of early human embryos. Now, you should realize, the reason in this previous slide that I went from a round circle representing a round ball of cells as an embryo, to no circle, is that everything I've told you until now, took place in a round ball of cells, OK? Up to about the 4, 00th cell stage, or about the 10, 00th cell stage, the frog embryo is just a round ball of cells, and most embryos are not very distinguished looking. But the process of making somites and dividing them, goes on until the embryo is a million cells or more, and during that time, the embryo reorganizes its body plan, so it's not longer a ball of cells, it starts to take on its characteristic form. And some of the characteristic form it takes on, is shown in this human embryo, about 19 days after fertilization, this region here is going to be the nervous system -- -- and on either side of the nervous system, I've got in red here, boxed these little segmented blocks, and you can see these segmented blocks in older embryos. Those are the somites from which most of the skeletal muscle is going to form. This is a movie to show you the segmentation of the somites. So here they go, here are the blocks of cells being divided one from another, it's a very beautiful process, and you can see it's very ordered. Down the middle here is the neural tube, which is starting to give rise to the central nervous system, and on either side of the neural tube is this future muscle tissue, or the future somites that include the skeletal muscle, and you can see them being divided symmetrically on either side of this midline here, in to these chunks of tissue. And it's actually a very interesting story in the regulation of gene expression, as to how you time things in such a coordinated fashion. In all animals, the somites become segmented first in the head region, and then the segmentation moves down towards the tail, and there is an intrinsic timer in the embryo, that I don't have time to describe to you, that again, converges on the regulation of gene expression, that allows this extraordinarily coordinated segmentation of the body plan. OK, so what I've done is to try and trace this ontology of the cell type. What you'll realize if you think about this, is that I haven't actually told you what transcription factors actually activate the myoD promoter in the somites. This is an RNA expression pattern, and the whole myoD regulatory loop, is controlled in a transcriptional way. And we don't know what exactly activates myoD expression in the somites, but we know a lot about what happens before. And with that, I am going to move on to what I want to spend most of today talking to you about, and that is the question of reproduction. And I'm going to talk to you about the molecular biology of reproduction, and particularly we're going to talk about sexual reproduction -- -- as one of the most important examples of cell-cell interactions. And in fact, the one without which none of us would be here, although that, in a sense, is a silly thing to say because without most of the interactions, the cell interactions during development, none of us would be here anyway. But, this is a very interesting process, a very important process, and it exemplifies some points that I want to bring up here. So the notion here is that two haploid cells, the sperm, and N is my designation for haploid, I presume you're all with me when I use N and 2N, as haploid and diploid, OK, fine. Sperm and egg go through the process of fertilization -- -- to form a single cell, a zygote that is diploid, and the zygote will then go on to perform a multi-cellular embryo, etc. Now, there are some really extraordinary things about this. One of the extraordinary things is that the sperm and the egg are fully differentiated cells. I have been telling you, indeed really trying to hammer into you, the notion that cells progress from less specialized states to more specialized states. And this is the one instance during development, where the flip is true, where the opposite is true. So the sperm and the egg are fully differentiated cells, they have reached this final cell type, but when they fuse, and they form the zygote, they now make the most fully undifferentiated cell. So the zygote is uncommitted, that may or may not be spelled correctly. So the zygote is uncommitted, and I want to introduce you to a new term, which is that of potency, where potency refers to the number of possible fates that a cell can assume. We're going to talk a lot about this when we talk about stem cells. The number of possible fates that a cell can assume, and the sperm and the egg are uni-potent, they have one possible fate, which is themselves. And the opposite is true of the zygote. It is totipotent, it can become everything, all possible cell types. And that is the antithesis of what happens during development in essentially, I would say, in every single cell type of the body. And it's extraordinary, and we don't understand, we don't understand, how you get this complete reversal of fates, although I'll try to go through this with you in a future lecture. All right, why go to all this trouble? This is a lot of work for an organism to go through, and the bottom line is that it leads to genetic re-assortment, and it leads to genetic diversity. So the notion of sexual reproduction, I'm going to write it up here, is that it engenders genetic diversity, and it does so because genetic materials becomes re-assorted during meiosis, and during recombination, or during the joining together, of the sperm and the egg. OK, I divided this lecture up into a few parts. The first is gametal genesis -- -- where the gametes are the egg and the sperm, just a different name for them, and gametogenesis is one of those developmental biology terms that has the suffix "-genesis", the creation of the gametes. Both the sperm and the egg start off from diploid, precursor cells, and this is a very interesting story, but I'm not going to tell you. But I'll remind you, well, I'm going to allude to it. I'll remind you that long ago, I showed you these beautiful series of pictures from worms. When we talked about determinants, cell autonomous factors that control cell fate, and I showed you how these determinants were segregated into a single cell, of the 32 cell worm embryo, and that this cell was going to give rise to the gametes, the egg and the sperm. In fact, the exact same thing is probably true in all animals, including ourselves. And certainly in frogs, and in fish, we know that there are determinants that are segregated, and we know what these are, they turn out to be proteins that bind RNA and control translation of presumably specific target genes. So, somewhere back in the dawn of time, during development, the germ cells become determined -- -- but I am not going to talk about this, I am going to talk about them when they actually already know that they're germ cells, and when they start thinking about assuming their final differentiated fate. And I want to make a couple of contrasting points, and OK, well let me do my board work and then we can look at that. OK. The precursor of the spermatozoa is the spermatagonium. This is a diploid cell that undergoes meiosis, to give rise to a haploid spermatazoan. The spermatagonium is a moderate sized cell, it's just a regular sized cell, and when it undergoes its conversion, its differentiation, to a spermatazoan, or a sperm, it becomes very small, it jettisons most of its cytoplasm, and it becomes motile. So you go from a medium sized cell to a tiny cell that has the very important property of being motile. And this is true, really, universally, some, and I'll talk about this in a moment. The way that spermatozoa are motile is slightly different in different organisms, but they pretty much, all spermatozoa are motile. In contrast, the egg arises from a diploid oogonium and the oogonium also starts off as a medium sized cell, but it does the opposite. It gets very big, OK? And the oogonium moves to change into something called an oocyte, or I prefer the term, egg, and this was a haploid cell. OK. It's large, by necessity sessile, and it's large because it stores a bunch of stuff, and the stuff that it stores is food, to nourish the early embryo before the embryo can fend for itself, or before it can be nourished by the mother. And it also contains components that regulate early development. So it's full of things like determinants. It's also full of machinery that can make ribosomes and so on, and the size of the egg varies from organism to organism. In frogs, for example, the egg is about a millimeter in diameter, in humans the egg is about 50 micrometers in diameter, because the mother nourishes the egg sooner than, or the embryo, sooner in humans than in frogs. All right, so let's look at a couple of these diagrams that I gave you. This is to remind you about meiosis and what meiosis is, and I want to make a couple of points that are up here on the top, some of which are more important than others. Spermatozoa, and spermatogenesis, is featured by continuing mitosis throughout life of the male. All the meiotic products become sperm, but this continuing mitosis is what's really important. The spermatogonium, the precursor of the spermatozoa, persists throughout life, and so you get a lot of sperm produced. So we can add to our list here, that you get many sperm. In humans there are about five times ten to the seventh sperm per ejaculate. If you go about five-fold lower than that, you're in the range of infertility. We can talk about why that is, and so one can do calculations, and it's been calculated that males can make up to ten to the 13th sperm in a lifetime. If you do the calculation, you will understand that not all of those sperm are used, and some are them are resorbed, so you can do that rather interesting calculation another time. OK. Oogenesis, in contrast to the plentiful and cheap sperm, and we'll come back to this point in a moment. In females, mitosis of the oogonia, ends before birth, and in fact, by the time a child is born, it is a female, it has about a million or so, primary oocytes, which are still diploid, they're along this pathway towards formation of the egg. And by the time the child is about five, there are only about 500, 00 primary oocytes sites. OK? And those cells have withdrawn from the cell cycle, they are not making anymore of them. So that is your pool from which to make eggs, and in fact, most of those, 90% of those, are going to die, and you're going to get a greater than 90% are going to die, and only about 500 eggs mature during the lifetime of a human female. OK? And it's actually not quite clear why, but it's certainly harder to make a good quality egg, probably, than to make lots of smaller sperm, for reasons of energy expenditure of the animal. All right, let's look through these slides a little more. The testes is an extraordinary organ that comprises many, many, many tubules, all coiled together. Within the seminiferous tubules there is an array of sperm production that is architecturally very interesting. Ignore this red up here for the moment, and look at this architecture of the wall of one of the The developing spermatozoa are embedded in cells called Sertoli cells. Sertoli cells supports, and nourish, and induce the formation of the mature spermatozoa. So the spermatozoa that are most immature, are lying away from the luminal, the cavity, of the seminiferous tubule, and as these cells mature and undergo meiosis, they move closer and closer to the lumen, until you get a bunch of spermatozoa, with these tails pointing out into the lumen of the tube, ready to be shed upon ejaculation. So it's a very beautifully, architecturally very beautiful process. Now what does the spermatazoan actually look like? It's got three parts. It's got this head that's got a nucleus that is highly condensed. I've told you previously that it's a real feat to pack the meter of DNA that's in every cell, into a little, tiny nucleus. You have to really pack it tightly. Well, it's even worse for a sperm because the nucleus of a sperm is an order of magnitude smaller, in many cases, than a somatic cell. So you have to pack the DNA even more tightly, and there are special proteins that overcome the charges on the DNA, to overcome the charge repulsion on the deoxyribonucleic acid, OK? So these are basic proteins that really pack the DNA tightly, to fit into this compact nucleus. The other thing we'll talk about, in a bit, is this structure called the acrosome. It derives from the Golgi, which you remember is involved in protein trafficking. Two other regions of the sperm that are almost universal, and very important, are this thing called the mid-piece, which is packed with mitochondria that are going to provide energy for the sperm as it moves. And finally, this tail region, which is a flagellum, now what's a flagellum? So, a flagellum is one of these biologically fantastic structures that uses polymers of proteins to make something that works in a concerted fashion. The proteins that it uses makes up the microtubules, so they are the tubulin proteins, we'll have more to say about them on Friday. We mentioned microtubules in the last lecture, in this case, microtubules pack together in a very ordered way, you can read this in your book. Microtubules pack together in a very ordered way, to make this very long structure, that when supplied with energy, will beat in a regular, often a sinusoidal fashion, and propel the cell forward, or wherever it is going. All right, female reproductive system. The ovary is the sight of egg production. In mammals, the uterus is the site of embryo growth, fertilization usually occurs somewhere between the ovary and the uterus, in the tube, the oviduct, or the fallopian tube, between the ovary and the uterus. All right, this is a diagram from your book again. In the developing, in the ovary, one can find eggs of developing, or oocytes sites of different stages, as they undergo development. They grow larger, and lie in a fluid-filled cavity, and eventually, I'll talk a bit more about this in a moment, this fluid filled cavity gets very large, and it bursts, and releases the egg. It's very interesting, in mammals, the egg is actually released almost into the body cavity, and it's gathered up by so called fimbrae, or folds of waving tissue that lie at the opening of the oviduct, or fallopian tube, and these kind of make a current that draws the released egg into the oviduct, so it can get on its way to the uterus. Subsequent to the egg being released, what's left of where the egg developed in the ovary, forms this thing called the corpus luteum, which secretes substances, hormones, that I'll talk about in a moment, that are very important for maintaining pregnancy, if this occurs. All right, you can see what I'm dwelling on here, not dwelling on. I'll dwell on things that I feel are more important, by writing things on the board, OK? So, stay with me. Let's go now to a system that I do want to dwell on more, which is the hormonal control of gametogenesis. Professor Jacks talked to you previously about hormones. Hormones are made in most animals, and their characteristic is that they work at very long range. So I want to characterize these as long-ranged, inducers, and they can be very long-range inducers. The hormones that control gametogenesis are made in the brain, in the hypothalamus, and the pituitary and they travel way through the bloodstream to get to the ovaries and the testes. This looks like a hermaphrodite here, [LAUGHTER], OK and this, I just realized this, OK, whatever. To get to the ovaries or the testes, or both, whatever, {LAUGHTER], OK, anyway, that point I want to make is that these hormones work at a very long distance. And I want to contrast that with the kinds of inducers that wee have been talking about in the preceding couple of lectures. The kind of classical, embryonic inducers, which act at very short range. So, for example, we talked about nodal signaling -- -- last lecture. For example, nodal, which is a ligand I mentioned, pivotal in making the mesoderm, nodal can only work over one, or a few, cell diameters. So if you were cells, you could have a conversation with one another, or maybe, the signal could get all the way to here, but it sure wouldn't get to the back of the room. Whereas, if you were a hormone, your signal would get all the way back to the back of the room, and probably all the way down the infinite corridor. OK, so there's a real order of magnitude different in the way hormones and other inducers can act. So, one to a few cells. All right, there are a bunch of different kinds of hormones that are involved in control of gametogenesis. I want to distinguish the steroid hormones, which are derivatives of cholesterol, and we mentioned them long ago in the lipid section. Steroids that include testosterone, estrogen, and progesterone, and act through a series of receptors that actually lies within the cell, they're not membrane-bound receptors, they are so-called nuclear receptors, so they act through nuclear receptors. I'm actually not going to write that on the board. And the other hormones I want to indicate are peptide hormones, that as their name indicates, are small polypeptide chains, and these include, I'm going to put abbreviations up, and you can get the full names later. FSH, LH, and GNRH, follicle-stimulating hormone, luteinizing hormone, and gonadotropin-releasing hormone. One of the things that is similar about the hormonal control of gametogenesis, to many other things we've been talking about, is the notion of feedback control. So, there is an enormous amount of feedback, both positive and negative in the circuitry that gives rise to the formation of egg and sperm. This is an example of the circuitry leading to stomatogenesis. Here is GNRH, released from the hypothalamus that stimulates the anterior pituitary, to produce lutenizing hormone, or follicle stimulating hormone. These act on cells within the testes, which act to, one set of cells acts to activate testosterone, the major male steroid hormone, and that acts back on the Sertoli cells in the seminiferous tubules, and together, these orchestrate the production of spermatozoa, and other various responses to these hormones. Now, the green indicates positive loops, and the red indicate negative feedback, so too much testosterone can have the effect of inhibiting the anterior pituitary's production of lutenizing hormone, or follicle-stimulating hormone. And there's a very careful balance of these hormones in the normal animal. Now, in females, things look similar. Again, GNRG, LH, and FSH, are produced to stimulate, release, and maturation of oocytes, and release from the ovary. The ovary, instead of producing testosterone, although it does produce some of that, produces estrogen and progesterone, and those are both required for normal maturation of the egg, and also for subsequent pregnancy. And also, as you will see, there is a feedback loop of both estrogen and progesterone, onto the hypothalamus and the anterior pituitary. And as this feedback loop that is exploited in the birth control pill, where if one gives high levels of estrogen and progesterone, you suppress the production of both GNRH from the hypothalamus, and LH and FSH from the anterior pituitary and therefore suppress the production of eggs from the ovary. Now actually looking, it's interesting that there's a female birth control pill, because really, there are certainly targets in the male for birth control pills, but these have not yet been exploited. But the notion is that you exploit this feedback loop, and its well-enough understood that you can. OK, so let me move on to talking about the process of fertilization, per say. The process of fertilization involves the egg and the sperm coming together, and recognizing one another as being from the same species. This is very important -- -- very important that you get species-specific recognition, in order that you get correct transmission of genetic material. So the first thing that we mention is sperm-egg recognition -- -- and this has many levels. In some animals, the egg, or the uterus, or the female sends out various chemo attractants to attractants, to encourage the sperm to get to the egg. Some of these have been isolated, and there are small peptides, for example, sea urchins, which are the organism that have been used to study fertilization. Most have very small peptides, or the chemo attractants, in humans there is some data that there may be chemo attractants released by the oviduct that causes the sperm to swim in a directed fashion, but it's very interesting that they can't be that powerful, those chemo attractants, because one needs so many sperm to be released in order to fertilize the egg. And it's very interesting that although there are five times ten to the seventh, or more, spermatozoa in a human ejaculate, if you actually look in the region of the oviduct, where fertilization would take place, you only find a few hundred sperm there. So most of them disappear on the way, and so if there are chemo attractants, I say they're not very good ones. OK, another process that is very important in mammals, is something called capacitation. The sperm, as I told you, have this capacity to be motile, that they contain a lot of energy stores, or they contain mitochondria that can convert energy to movement. But they save that energy for a time when they think they might be in the vicinity of an egg, and when sperm are exposed to fluid from a mammalian oviduct, they undergo an increase in motility, so capacitation leads to an increase in sperm motility. This process is mediated by some kind of signal transduction mechanism, that involves the second messenger, cyclic AMP that you discussed with Professor Jacks, and this is very important. If capacitation doesn't occur, fertilization doesn't occur either. OK, so once the sperm are in the vicinity of the egg, they then have the challenge of fusing with it. This is an animation I showed you previously, of a sperm moving towards an egg. Now this is a mammalian egg, and it's surrounded by a bunch of stuff. It's surrounded by these circly things, which are cells that released from the ovary, along with the egg, and it's surrounded by a number of so-called membranes. The most important one of which is called the zona pellucida, and as the sperm goes towards the egg, and approaches the egg, it has to burrow its way through a bunch of membranes to get there, this animation doesn't finish fertilization clearly. OK, so if you look at a mammalian egg at fertilization, there is the egg per-say, that is a cell surrounded by a plasma membrane, and then there's this thick, yellow layer, that's called the zona pellucida. Around that, is this layer of cells called the cumulus, and the sperm have to borrow through both the cumulus layer, and particularly the zona pellucida, before they get to the plasma membrane, and before they can then try to fuse with the plasma membrane, and enter the egg. So, the second thing that we need to think about is contact, and there's two steps to this. The first contact and they're actually very interesting steps because there's a bunch of reciprocal interaction, and reciprocal receptor ligand interaction. The first interaction is between the sperm, which provides a receptor, and the egg, and this layer called the zona pellucida around the egg, and the zona provides the ligands for the interaction. And it turns out that the zona pellucida, although it's called a membrane very often, is not a lipid by-layer, it's a glycol-protein layer, OK? And the proteins in the zona are called zp1, zp2, and zp3, and they form a very complex meshwork of glycol-proteins. The sperm receptor in mammals recognizes a protein called zp3 in the zona, and then subsequently, there's a shift in carbohydrate arrangement of the zona proteins, and it then recognizes something called zp2. Now, this receptor-ligand interaction has a very interesting consequence. It leads to activation of second messengers, it goes through a system of proteins called G proteins, but what was most important is that it leads to the release of calcium within the sperm. And this calcium, let me move the calcium here, so this leads to the release of calcium with the sperm, and this causes something called the acrosome reaction. Now the acrosome, as I said, derives from the Golgi, and it is filled with Golgi-derived proteins, particularly proteases. Upon the acrosome reaction, there is a massive exocytosis of the acrosomal contents, to release proteases, and these proteases digest the zona. All right, some diagrams. This is a diagram you have on your handout. Here is the sperm moving through the cumulus layer, getting through the zona, at contact there is a reaction, such that the acrosome releases its contents, and the next step can happen. This is a movie of the acrosome reaction from the horseshoe crab, limulus, it has an extraordinary acrosome. The acrosome in limulus compromises acrosomal protein, the proteases, but a lot of unpolymerized, and upon contact with the limulus egg, the, let me stop this so you can watch it while I'm telling you about it. The actin that is in the acrosome polymerizes, this is a matter of seconds, and sends out this incredibly long process, here it comes, look at this. See this thing shooting out? That is a polymer of actin that is coated with these acrosomal proteases. So this is the sperm head, and so it's shooting out this process that is many, many times longer than the sperm head, and it's allowing it to get into the limulus egg. Mammalian sperm do not have such a spectacular acrosome reaction, but they do have one that serves the same purpose. OK, all right, so the next thing that is going to happen is that there is a second reciprocal interaction, and this time, the egg is going to provide the receptor, and the sperm is going to provide the ligand. So, we now have the sperm, whose acrosomal membrane that had been the Golgi membrane, is now exposed, and is covered with ligands, so the acrosome, the acrosome membrane, is covered with ligand. This interacts with receptors on the egg plasma membrane, and there is, as a result of this receptor-ligand interaction, the activation of a signal-transduction system, and the signal transduction system does two things, so there is this interaction leads within the egg, to activation of signal transduction, that has the effect, eventually, of releasing calcium with the egg. And this calcium released within the egg does two things. The first thing that it does is to prevent any additional sperm from entering the egg, and I'll tell you about this in a second. So it blocks, or activates the block, to polyspermy, and in particular it activates the so-called slow block to polyspermy, and it also activates, a little while later, the zygote, to begin dividing, to enter the cell cycle, and to begin various other processes required in the early embryo. So cell cycle entry of the zygote. All right, so let's look at a movie of the sperm entering the egg. OK, here is, I'll show you this again. Here is the sperm, its tale is sticking out, there, it's going right into the egg. The cell membrane reciprocates, and buckles, and in some organisms, it engulfs the sperm as it's going in, but the actual sense in most organisms, the membranes either fuse, or somehow you get the whole sperm being pushed into the egg. In most animals the sperm membrane doesn't actually enter the egg, but in some animals it does, and in some cases it doesn't seem to matter. OK, what is this block to polyspermy? This is something that happens in the first 30 seconds after fertilization, and it involves exocytosis of a set of granules called the cortical granules, that contain enzymes, that do two things. These enzymes firstly, when they are released, break apart junctions between the outer membranes, in this case, this is shown in frogs. The outer membrane in frogs is called the vitelline envelope, its equivalent to the zona pellucida in mammals. And before fertilization, this outer envelope is tethered by proteins, to the plasma membrane, so they're very closely opposed. At the release of the cortical granules, these protein contacts are broken, and the fertilization envelope lifts off the surface of the egg. A number of other things happen, as well. In mammals the zona pellucida is completely remodeled, so it no longer binds with the sperm, the carbohydrates are stripped off the zona proteins so that they no longer bind sperm. This is a movie of the block to polyspermy, or the consequence of the block to polyspermy in the frog. Here is the frog egg. At fertilization, watch carefully, you can see there is a big halo that arises above the frog egg, there it is, and this is a consequence of cortical granule exocytosis, and the blocked polyspermy. OK. OK. Before you go, what I did not cover in lecture today, don't go for one second.