Flash and JavaScript are required for this feature.
Download the video from iTunes U or the Internet Archive.
Topics covered: Exceptions to Lewis structure rules; Ionic bonds
Instructor: Catherine Drennan, Elizabeth Vogel Taylor
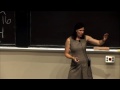
Lecture 12: Ionic Bonds
Related Resources
Lecture Notes (PDF - 1.1MB)
The following content is provided under a Creative Commons license. Your support will help MIT OpenCourseWare continue to offer high quality educational resources for free. To make a donation or view additional materials from hundreds of MIT courses, visit MIT OpenCourseWare at ocw.mit.edu.
PROFESSOR: OK, let's get started here. Go ahead and take 10 more seconds on the clicker question, which probably looks all too familiar at this point, if you went to recitation yesterday. All right, and let's see how we do here.
OK. So, let's talk about this for one second. So what we're asking here, if we can settle down and listen up, is which equations can be used if we're talking about converting wavelength to energy for an electron. Remember, the key word here is electron. This might look familiar to the first part of problem one on the exam, and problem one on the exam is what tended to be the huge problem on the exam. I think over 2/3 of you decided on the exam to use this first equation, e equals h c over wavelength.
So I just want to reiterate one more time, why can we not use this equation if we're talking about an electron? C. OK, good, good, I'm hearing it. So the answer is c. What you need to do is you need to ask yourself if you're trying to convert from wavelength to energy for an electron, and you are tempted, because we are all tempted to use this equation, and if you were tempted, say, does an electron travel at the speed of light? And if the answer is no, an electron does not travel at the speed of light, light travels at the speed of light, then you want to stay away from using this equation. And I know how tempting it is to do that, but we have other equations we can use -- the DeBroglie wavelength, and this is just a combination of energy equals 1/2 m v squared, and the definition of momentum, so we can combine those things to get it.
You might be wondering why I'm telling you this now, you've already -- if you've lost points on that, lost the points on it, and what I'm saying to you is if there are parts of exam 1 that you did not do well on, you will have a chance to show us again that you now understand that material on the final. One quarter of the final is going to be exam 1 material, and what that means is when we look at your grade at the end of the semester, and we take a look at what you got on exam 1, and you're right at that borderline, and we say well, what happened, did they understand more at the end of the semester, did the concepts kind of solidify over the semester? And if they did and if you showed us that they did, then you're going to get bumped up into that next grade category.
So keep that in mind as you're reviewing the exam, sometimes if things don't go as well as you want them to, the temptation is just to put that exam away forever and ever. But the reality is that new material builds on that material, and specifically exam 1 a, question 1 a that deals with converting wavelength to energy for an electron. I really want you guys know this and to understand it, so I can guarantee you that you will see this on the final. Specifically, question 1, part a. You will see something very, very similar to this on the final. If you are thinking about 1 thing to go back and study on exam 1, 1 a is a really good choice for that. This is important to me, so you're going to see it on the final.
So if you have friends that aren't here, you might want to mention it to them, or maybe not, maybe this is your reward for coming to class, which is fine with me as well.
All right. So I want to talk a little bit about exam 1. I know most you picked up your examine in recitation. If you didn't, any extra exams can be picked up in the Chemistry Education office, that's room 2204.
So, the class average for the exam was a 68%, which is actually a strong, solid average for an exam 1 grade in the fall semester of 511-1. What we typically see is something right in this range, either ranging from the 50's for an exam 1 average to occasionally getting into the 70's, but most commonly what we've seen for exam 1 averages is 60, 61 -- those low 60's. So in many ways, seeing this 68 here, this is a great sign that we are off to a good start for this semester. And I do want to address, because I know many of you, this is only your second exam at MIT, and perhaps you've never gotten an exam back that didn't start with a 90 or start with an 80 in terms of the grades. So one thing you need to keep in mind is don't just look at the number grade. The reason that we give you these letters grade categories is that you can understand what it actually means, what your exam score actually says in terms of how we perceive you as understanding the material.
So, for example, and this is the same categories that were shared in recitation, so I apologize for repeating, but I know sometimes when you get an exam back, no more information comes into your head except obsessing over the exam, so I'm just going to say it one more time, and that is between 88 and 100, so that's 20% of you got an A. This is just absolutely fantastic, you really nailed this very hard material and these hard questions on the exam where you had to both use equations and solve problems, but also understand the concept in order to get yourself started on solving the problem.
The same with the B, the B range was between 69 and 87 -- anywhere in between those ranges, you've got a B, some sort of B on the exam. So again, if you're in the A or the B category here, this is really something to be proud of, you really earned these grades. You know these exams, our 511-1 exams, we're not giving you points here, there are no give me, easy points, you earned every single one of these points. So, A and B here, these are refrigerator-worthy grades, hang those up in your dorm. This is something to feel good about.
All right. So, for those of you that got between a 51 and a 68, this is somewhere in the C range. For some people, they feel comfortable being in the C range, other people really do not like being in this range. We understand that, there is plenty of room up there with the A's and the B's. You are welcome to come up to these higher ranges starting with the next exam. And what I want to tell you if you are in the C range, and this is not a place that you want to be in, anyone that's got below the class average, so below a 68 -- or a 68 or below, is eligible for free tutoring, and I put the website on the front page of your notes. This means you get a one-on-one tutor paid for by the Chemistry Department to help you if it's concepts you're not quite up on, if it's exam strategy that you need to work on more. Whatever it is that you need to work on, we want to help you get there.
So, if you have a grade that you're not happy with, that you're feeling upset or discouraged about, please, I'm happy to talk to all of you about your grades individually. You can come talk to me, bring your exam, and we'll go over what the strategy should be in terms of you succeeding on the next exam. You can do the same thing with all of your TAs are more than happy to meet with each and every one of you. And then in addition to that, we can set you up with a tutor if you are in the C range or below, in terms of this first exam.
All right. So 44 to 50, this is going to be in the D range. And then anything below a 44 is going to be failing on this exam. And also keep in mind, for those of you that are freshman, you need at least a C to pass the class. So, if you did get a D or an F on the first exam, you are going to need to really evaluate why that happened and make some changes, and we're absolutely here to help you do that. So the real key is identifying where the problem is -- is it with understanding the concepts, are you in a study group that's dragging you along but you're not understanding? Do you kind of panic when you get in the exam? There are all sorts of scenarios we can talk about and we want to talk about them with you.
Seriously, even if we had a huge range in this exam from 17 to 100, if you're sitting there and you're the 17, and actually there's more than 1 so don't feel alone, if you're a 17 or you're a 20, it's not time to give up, it's not time to drop the class and say I'm no good at chemistry, I can't do this. You still can, this is your first couple of exams, certainly your first in this class, potentially one of your first at MIT, so there's tons of room to improve from here on out. This is only 100 points out of 750. So, the same thing goes if you did really well, you still have 650 other points that you need to deal with. So, make sure you don't just rest on your high score from this first exam.
So, OK, so that's pretty much what I wanted to say about the exam, and in terms of there's tons of resources if things didn't work out quite as you wanted. If you feel upset in any way, please come and talk to me. We want you to love chemistry and feel good about your ability to do it. Nobody get into MIT by mistake, so you all deserve to be sitting here, and you all can pass this class and do well in it, so we can help you get there no matter what. You all absolutely can do this.
And then one more time, to reiterate, in case anyone missed it, 1 a, make sure you understand that, I feel like that's important. And actually all of 1 -- I really feel like the photoelectric effect is important for understanding all of these energy concepts. So, as you go on in this class, make sure you don't go on before you go back and make sure you understand that problem.
All right, so let's move on to material for exam 2 now, and we're already three lectures into exam 2 material. And I do want to say that in terms of 511-1, what tends to happen is the exam scores go up and up and up, in terms of as we go from exam 1, to exam 2, to exam 3. One of these reasons is we are building on material, the other reason is you'll be shocked at how much better you are at taking an exam just a few weeks from now. So this will be on, starting with the Lewis structures, so go back in your notes -- if this doesn't sound familiar, if you spent too much time -- or not too much time, spent a lot of time studying exam 1 and didn't move on here.
Today we're going to talk about the breakdown of the octet rule. Cases where we don't have eight electrons around our Lewis structures, then we'll move on to talking about ionic bonds. We had already talked about covalent bonds, and then we talked about Lewis structures, which describe the electron configuration in covalent bonds. So now let's think about the other extreme of ionic bonds, and then we'll talk about polar covalent bonds to end, if we get there or will start with that in class on Monday.
Also, public service announcement for all of you, voter registration in Massachusetts, which is where we are, is on Monday, the deadline if you want to register to vote. There's some websites up there that can guide you through registering and also can guide you through, if you need an absentee ballot for your home state. And I actually saw, and I saw a 5.111 student manning, there's some booths around MIT that will register you or get you an absentee ballot. So, the deadline's coming soon, so patriotic duty, I need to remind you of that as your chemistry teacher -- chemistry issues are important in politics as well. So make sure you get registered to vote.
I just remembered one more announcement, too, that I did want to mention, some of you may have friends in 511-2 and have heard their class average for exam 1. And I want to tell you, this happens every year, their average was 15 points higher than our average. Last year, their average was 15 points higher than our average. This is for exam 1. This is what tends to happen to 511-2 grades as the exam goes on. This is what happens to 511-1. You guys are in a good spot. Also, I want to point out that what's not important is just that number grade, but also the letter that goes with it.
So, for example, if you got a 69 in this class on this exam, that's a B minus. If you got a 69 on your exam in 511-2, that's a D, you didn't pass the exam. So keep that in mind when your friend might have gotten a higher number grade than you and you know you understand the similar material just as well. Similarly, an 80 in this class on the exam was a B plus, a very high B. An 80 in that class is going to be a C. So, just don't worry so much about exactly where that average lies, you really want to think about what the letter grade means. OK, I've said enough. I just -- I hate to see people discouraged, and I know that a few people have been feeling discouraged, so that's my long-winded explanation of exam 1 grades.
All right. So, let's move on with life though, so talking about the breakdown of the octet rule. The first example where we're going to see a breakdown is any time we have an odd number of valence electrons. This is probably the easiest to explain and to think about, because if we have an odd number that means that we can't have our octet rule, because our octet rule works by pairing electrons. And if we have an odd number, we automatically have an odd electron out.
So, if we look at an example, the methyl radical, we can first think about how we draw the Lewis structure -- we draw the skeletal structure here. And then what we're going to do is add up our valence electrons -- we have 3 times 1 for the hydrogen atoms, carbon has 4 valence electrons, so we have a total of 7. If we want to fill all of our valence shells in each of these atoms, we're going to need a total of 14 electrons. So, what we see we're left with is that we have 7 bonding electrons. So we can fill in 6 of those straightforward here, because we know that we need to make 3 different bonds. And now we're left over with 1 electron, we can't make a bond.
So, what we'll do is carbon does not have an octet yet. We can't get it one, but we can do the best we can and help it out with adding that extra electron onto the carbon atom, so that at least we're getting as close as possible to filling our octets.
This is what we call a radical species or a free radical. Free radical or radical species is essentially any type of a molecule that has this unpaired electron on one of the atoms. This might look really strange, we're used to seeing octets. But you'll realize, if you calculate the formal charge on this molecule, that it's not the worst situation ever for carbon. At least it's formal charge is zero, even if it doesn't have -- it would rather have an extra bond and have a full octet. But it's not the worst scenario that we can imagine. But still, radicals tend to be incredibly reactive because they do want to fill that octet.
So, what happens when you have a radical is it tends to react with the first thing that it runs into, especially highly reactive radicals that are not stabilized in some other way, which you'll tend to talk about it organic chemistry -- how you can stabilize radicals.
So the term free radical should sound familiar to you, whether you've heard it in chemistry before, or you haven't heard it in chemistry, but maybe have heard it, I don't know, commercials for facial products or other things. People like to talk about free radicals, and they're sort of the hero that gets rid of free radicals, which are antioxidants. So you hear in a lot of different creams or products or vitamins that they have antioxidants in them, which get rid of free radicals. The reason you would want to get rid of free radicals is that free radicals can damage DNA, so they're incredibly reactive. It makes sense that if they hit a strand of DNA, they're going to react with the DNA, you end up breaking the strands of DNA and causing DNA damage.
So, this is actually what happens in aging because we have a lot of free radicals in our body. We can introduce them artificially, for example, cigarette smoke has a lot of really dangerous free radicals that get into the cells in your lungs, which damage your lung DNA, which can cause lung cancer. But also, all of us are living and breathing, which means we're having metabolism go on in our body, which means that as we use oxygen and as we metabolize our food, we are actually producing free radicals as well. So it's kind of a paradox because we need them because they are a natural by-product of these important processes, but then they can go on and damage cells, which is what kind of is causing aging and can lead to cancer.
We have enzymes in our body that repair damage that is done by free radicals, that will put the strands of DNA back together. And we also have antioxidants in our body. So, you might know that, for example, very brightly colored fruit is full of antioxidants, they're full of chemicals that will neutralize free radicals. Lots of vitamins are also antioxidants, so we have vitamin A on the top there and vitamin E.
So, the most common thing we think of when we think of free radicals is very reactive, bad for your body, causes DNA damage. But the reality is that free radicals are also essential for life. So this is kind of interesting to think about. And, for example, certain enzymes or proteins actually use free radicals in order to carry out the reactions that they carry out in your body. So, for example, this is a picture or a snapshot of a protein, this is a crystal structure of ribonucleotide reductase is what it's called. It's an enzyme that catalyzes the reaction of an essential step in both DNA synthesis and also DNA repair, and it requires having radicals within its active site in order to carry out the chemistry.
So, this is kind of a neat paradox, because radicals damage DNA, but in order to repair your DNA, you need certain enzymes, and those enzymes require different types of free radicals. So, free radicals are definitely very interesting, and once we get -- or hopefully you will get into organic chemistry at some point and get to really think about what they do in terms of a radical mechanism.
We can think about radicals that are also more stable, so let's do another example with the molecule nitric acid. So we can again, draw the skeleton here, and just by looking at it we might not know it's a radical, but as we start to count valence electrons, we should be able to figure it out very quickly, because what we have is 11 valence electrons. We need 16 electrons to have full octets. So, we're left with 5 bonding electrons. We put a double bond in between our nitrogen and our oxygen, so what we're left over with is this single bonding electron, and we'll put that on the nitrogen here. And I'll explain why we put it on the nitrogen and not the oxygen in just a minute.
But what we find is then once we fill in the rest of the valence electrons in terms of lone pairs, this is the structure that we get. And if you add up all of the formal charges on the nitrogen and on the oxygen, what you'll see is they're both 0. So if you happen to try drawing this structure and you put the lone pair on oxygen and then you figured out the formal charge and saw that you had a split charge, a plus 1 and a minus 1, the first thing you might want to try is putting it on the other atom, and once you did that you'd see that you had a better structure with no formal charge.
I have to mention what nitric oxide does, because it's a very interesting molecule. Don't get it confused with nitrous oxide, which is happy gas, that's n o 2. This is nitric oxide, and it's actually much more interesting than nitrous oxide. It's a signaling molecule in your body, it's one of the very few signaling molecules that is a gas, and obviously, it's also a radical. What happens with n o is that it's produced in the endothelium of your blood vessels, so the inner lining of your blood vessels, and it signals for smooth muscle that line your blood vessels to relax, which causes vasodilation , and by vasodilation, I just mean a widening of the blood vessels. So, n o signals for your blood vessels to get wider and allow more blood to flow through. And if you think about what consequences this could have, in terms of places where they have high altitude, so they have lower oxygen levels, do you think that they produce more or less and n o their body? More? Yeah, it turns out they do produce more. The reason they produce more is that they want to have more blood flowing through their veins so that they can get more oxygenated blood into different parts of their body.
N o is also a target in the pharmaceutical industry. A very famous one that became famous I guess over 10 years ago now, and this is from a drug that actually targets one of n o's receptors, and this drug has the net effect of vasodilation or widening of blood vessels in a certain area in the body. So this is viagra, some of you may be familiar, I think everyone's heard of viagra. Now you know how viagra works. Viagra breaks down, or it inhibits the breakdown of n o's binding partner in just certain areas, not everywhere in your body. So, in those areas, what happens is you get more n o signaling, you get more vasodilation, you get increased blood flow. So that's a little bit of pharmacology for you here today.
All right, so let's talk about one more example in terms of the breakdown of the octet rule with radicals. Let's think about molecular oxygen. So let's go ahead and quickly draw this Lewis structure. We have o 2. The second thing we need to do is figure out valence electrons. 6 plus 6, so we would expect to see 12. For a complete octet we would need 8 electrons each, so 16. So in terms of bonding electrons, what we have is 4 bonding electrons. So, we can go ahead and fill those in as a double bond between the two oxygens.
So, what we end up having left, and this would be step six then because five was just filling in that, is 12 minus 4, so we have 8 lone pair electrons left. So we can just fill it in to our oxygens like this.
All right, so using everything we've learned about Lewis structures, we here have the structure of molecular oxygen. And I just want to point out for anyone that gets confused, when we talk about oxygen as an atom, that's o, but molecular oxygen is actually o 2, the same for molecular hydrogen, for example.
All right, so let's look at what the actual Lewis structure is for molecular oxygen, and it turns out that actually we don't have a double bond, we have a single bond, and we have two radicals. And any time we have two radicals, we talk about what's called a biradical. And while using this exception to the Lewis structure rule, to the octet rule for odd numbers of valence electrons can clue us into the fact that we have a radical, there's really no way for us to use Lewis structures to predict when we have a biradical, right, because we would just predict that we would get this Lewis structure here.
So, when I first introduced Lewis structures, I said these are great, they're really easy to use and they work about 90% of the time. This falls into that 10% that Lewis structures don't work for us. It turns out, in order to understand that this is the electron configuration for o 2, we need to use something called molecular orbital theory, and just wait till next Wednesday and we will tell you what that is, and we will, in fact, use it for oxygen. But until that point, I'll just tell you that molecular orbital theory takes into account quantum mechanics, which Lewis theory does not. So that's why, in fact, there are those 10% of cases that Lewis structures don't work for.
All right, the second case of exceptions to the octet rule are when we have octet deficient molecules. So basically, this means we're going to have a molecule that's stable, even though it doesn't have a complete octet. And these tend to happen in group 13 molecules, and actually happen almost exclusively in group 13 molecules, specifically with boron and aluminum. So, any time you see a Lewis structure with boron or aluminum, you want to just remember that I should look out to make sure that these might have an incomplete octet, so look out for that when you see those atoms.
So, let's look at b f 3 as our example here. And what we see for b f 3 is the number of valence electrons that we have are 24, because the valence number of electrons for boron is 3, and then 3 times 7 for each fluorine. For total filled octets we need 32, so that means we need 8 bonding electrons. So, let's assign two to each bond here, and then we're going to have two extra bonding electrons, so let's just arbitrarily pick a fluorine to give a double bond to. And then we can fill in the lone pair electrons, we have 16 left over. So thinking about what the formal charge is, if we want to figure out the formal charge for the boron here, what we're talking about is the valence number for boron, which is 3, minus 0 because there are no lone pairs, minus 1/2 of 8 because there are eight shared electrons. We get a formal charge of minus 1.
What is our formal charge since we learned this on Monday for thinking about the double bonded fluorine in boron? So, look at your notes and look at the fluorine that has a double bond with it, and I want you to go ahead and tell me what that formal charge should be.
All right, let's take 10 more seconds on that. OK, so 49%. So, let's go look back at the notes, we'll talk about why about 50% of you are right, and 50% need to review, which I totally understand you haven't had time to do yet, your formal charge rules from Monday's class, there were other things going on. But let's talk about how we figure out formal charge. Formal charge is just the number of valence electrons you have. So fluorine has 7. You should be able to look at a periodic table and see that fluorine has seven. What we subtract from that is the number of lone pair electrons, and there are four lone pair electrons on this double bonded fluorine, so it's minus 4. Then we subtract 1/2 of the shared electrons. Well we have a double bond with boron here, so we have a total of 4 shared electrons. And when we do the subtraction here, what we end up with is a formal charge plus 1 on the double bonded fluorine.
Without even doing a calculation, what do you think that the formal charge should be on you single bonded fluorines? Good. OK, it should be 0 and it is 0. The reason it's zero in terms of calculating it is 7 minus 6 lone pair electrons minus 1/2 half of 2 shared electrons is 0. The reason that you all told me, I think, and I hope, is that you know that the formal charge on individual atoms has to equal the total charge on the molecule. So if we already have a minus 1 and a plus 1, and we know we have no charge in the molecule, and we only have one type of atom left to talk about, that formal charge had better be 0.
OK. So this looks pretty good in terms of a Lewis structure, we figured out our formal charges. These also look pretty good, too, we don't have too much charge separation. But what actually it turns out is that if you experimentally look at what type of bonds you have, it turns out that all three of the b f bonds are equal in length, and they all have a length that would correspond to a single bond. So, experimentally, we know we have to throw out this Lewis structure here, we have some more information, let's think about how this could happen.
So this could happen, for example, is if we take this two of the electrons that are in the b f double bond and we put it right on to the fluorine here, so now we have all single bonds. And let's think about what the formal charge situation would be in this case here. What happens here is now we would have a formal charge of 0 on the boron, we'd have a formal charge of 0 on all of the fluorine molecules as well. So, it turns out that actually looking at formal charge, even though the first case didn't look too bad, this case actually looks a lot better. We have absolutely no formal charge separation whatsoever. It turns out again, boron and aluminum, those are the two that you want to look out for. They can be perfectly happy without a full octet, they're perfectly happy with 6 instead of 8 in terms of electrons in their valence shell. So that is our exception the number two.
We have one more exception and this is a valence shell expansion, and this can be the hardest to look out for, students tend to forget to look for this one, but it's very important as well, because there are a lot of structures that are affected for this . And this is only applicable if we're talking about a central atom that has an n value or a principle quantum number that's equal to or greater than three. What happens when we have n that's equal to or greater to three, is that now, in addition to s orbitals and p orbitals, what else do we have available to us? D orbitals, great. So what we see is we have some empty d orbitals, which means that we can have more than eight electrons that fit around that central atom.
If you're looking to see if this is going to happen, do you think this would happen with a large or small central atom? So think of it in terms of just fitting. We've got to fit more than 8 electrons around here. Yeah, so it's going to be, we need to have a large central atom in order for this to take place. Literally, we just need to fit everything around is probably the easiest way to think about it. And what happens is it also tends to have small atoms that it's bonded to. Again, just think of it in terms of all fitting in there.
So, let's take an example p c l 5. The first example is the more straightforward example, because let's start to draw the Lewis structure, and what we see is that phosphorous has five chlorines around it. So we already know if we want to form five bonds we've broken our octet rule. But let's go through and figure this out and see how that happens.
What we know is we need 40 valence electrons, we have those -- 5 from the phosphorous, and we have 7 from each of the chlorine atoms. If we were to fill out all of those octets, that would be 48 electrons. So what we end up with when we do our Lewis structure calculation is that we only have 8 bonding electrons available to us. So we can fill those in between the phosphorous and the chlorine, those 8 bonding electrons.
So, this is obviously a problem. To make 5 p c l bonds we need 10 shared electrons, and we know that that's the situation because it's called p c l 5 and not p c l 4, so we can go right ahead and add in that extra electron pair. So we've used up 10 for bonding, so that means what we have left is 30 lone pair electrons, and I would not recommend filling all of these in your notes right now, you can go back and do that, but just know the rest end up filling up the octets for all of the chlorines.
So, in this first case where you actually need to make more than for bonds, you will immediately know you need to use this exception to the Lewis structure octet rule, but sometimes it won't be as obvious. So, let's look at c r o 4, the 2 minus version here, so a chromate ion, and if we draw the skeletal structure, we have four things that the chromate needs to bond to.
So, let's do the Lewis structure again. When we figure out the valence electrons, we have total, we have 6 from the chromium, we have 6 from each of the different oxygens, and where did this 2 come from? Yup, the negative charge. So, remember, we have 2 extra electrons hanging out in our molecule, so we need to include those. We have a total of 32. 40 are needed to fill up octets. So again, we have 8 bonding electrons available, so we can go ahead and fill these in between each of the bonds. What happens is that we then have 24 lone pair electrons left, and we can fill those in like this. And the problem comes now when we figure out the formal charge.
So, when we do that what we find is that the chromium has a formal charge of plus 1, and that each of the oxygens has a total charge of minus 1. So we actually have a bit of charge separation here. Without even doing a calculation, what is the total charge of these that are added up? OK, it's minus 2, that's right. We know that the total charge of each of the formal charges has to add up to minus 2, because that's the charge in our molecule. We can also just calculate it -- the chromate gives us a plus 2, then we have 4 times minus 1 for each of the oxygens, so we have a minus 2.
So, we have some charge separation here, and in some cases, if we're not at n equals 3 or higher, there's really nothing we can do about it, this would be the best structure we can do. But since we have these d orbitals available, we can use them, and it turns out that experimentally this is what's found, that the length and the strength are not single bonds, but they're actually something between a single bond and a double bond.
So how do we get a 1 and 1/2 bond, for example, what's the term that let's us do that? Resonance. That's right. So that's exactly what's happening here. So, if we went ahead and drew this structure here where we have now two double bonds and two single bonds, that would be in resonance with another structure where we have two double bonds instead to these two oxygens, and now, single bonds to these two oxygens. We can actually also have several other resonance structures as well. Remember, the definition of a resonance structure is where all the atoms stay the same, but what we can do is move around the electrons -- we're moving around those extra two electrons that can be in double bonds.
So, why don't you tell me how many other resonance structures you would expect to see for this chromate ion? All right, let's take 10 more seconds on this.
All right. This is good. I know this is a real split response, but the right answer is the one that is indicated in the graph here that it's four. This takes a little bit of time to get used to thinking about all the different Lewis structures you can have. So, you guys should all go back home if you can't see it immediately right now and try drawing out those four other Lewis structures, for chromate, there are four others. You'll probably get a chance to literally do this example in recitation where you draw out all four, but it's even better to make sure you understand it before you get to that point. So, we can go back to the class notes.
So it turns out there's four other Lewis structures, so basically just think about all the other different combinations where you can have single and double bonds, and when you draw those out, you end up with four. So, for every single one of these Lewis structures, we could figure out what the formal charges are, and what we would find is that it's 0 on the chromium, it's 0 for the double bonded oxygens, and it's going to be negative 1 for the single bonded oxygens.
So, what you can see is that in this situation, we end up having less formal charge separation, and that's what we're looking for, that's the more stable structure. So any time you can have an expanded octet -- an expanded valence shell, where you have n is equal to or greater than 3, and by expanding and adding more electrons into that valence shell, you lower the charge separation, you want to do that.
I also want to point out, I basically said there's 6 different ways we can draw this in terms of drawing all the resonance structures. You might be wondering if you have to figure out the formal charge for each structure individually, and the answer is no, you can pick any single structure and the formal charges will work out the same. So, for example, if you pick this structure and your friend picks this structure, you'll both get the right answer that there's just the negative 1 on the oxygens and no other formal charges in the molecule.
All right. So those are the end of our exceptions to the octet rule for Lewis structures, that's everything we're going to say about Lewis structures. And remember, that when we talk about Lewis structures, what they tell us is the electron configuration in covalent bonds, so that valence shell electron configuration. So we talked a lot about covalent bonds before we got into Lewis structures, and then how to represent covalent bonds by Lewis structures.
So now I'll say a little bit about ionic bonds, which are the other extreme, and when you have an ionic bond, what you have now is a complete transfer of either one or many electrons between two atoms. So the key word for covalent bond was electron sharing, the key word for ionic bonds is electron transfer. And the bonding between the two atoms ends up resulting from an attraction that we're very familiar with, which is the Coulomb or the electrostatic attraction between the negatively charged and the positively charged ions.
So let's take an example. The easiest one to think about is where we have a negative 1 and a positive 1 ion. So this is salt, n a c l -- actually lots of things are call salt, but this is what we think of a table salt. So, let's think about what we have to do if we want the form sodium chloride from the neutral sodium and chlorine atoms. So, the first thing that we're going to need to do is we need to convert sodium into sodium plus.
What does this process look like to you? Is this one of those periodic trends, perhaps? Can anyone name what we're looking at here? Exactly, ionization energy. So, if we're going to talk about the energy difference here, what we're going to be talking about is the ionization energy, or the energy it takes to rip off an electron from sodium in order to form the sodium plus ion. So, we can just put right here, that's 494 kilojoules per mole.
The next thing that we want to look at is chlorine, so in terms of chlorine we need to go to chlorine minus, so we actually need to add an electron. This is actually the reverse of one of the periodic trends we talked about. Which trend is that this is the reverse of? Electron affinity, right. Because if we go backwards we're saying how badly does chlorine want to grab an electron? Chlorine wants to do this very badly, and it turns out the electron affinity for chlorine is huge, it's 349 kilojoules per mole, but remember, we're going in reverse, so we need to talk about it as negative 349 kilojoules per mole.
So if we talk about the sum of what's happening here, what we need to do is think about going from the neutrals to the ions, so we can just add those two energies together, and what we end up with is plus 145 kilojoules per mole, in order to go from neutral sodium in chlorine to the ions.
So, the problem here is that we have to actually put energy into our system, so this doesn't seem favorable, right. What's favorable is when we actually get energy out and our energy gets lower, but what we're saying here is that we actually need to put in energy. So another way to say this is this process actually requires energy. It does not emit energy, it does not give off excess energy, it requires energy.
So, we need to think about how can we solve this problem in terms of thinking about ionic bonds, and the answer is Coulomb attraction. So there's one more force that we need to talk about, and that is when we talk about the attraction between the negatively and the positively charged ions, such that we form sodium chloride. So this process here has a delta energy, a change in energy of negative 589 kilojoules per mole. So that's huge, we're giving off a lot of energy by this attraction. So if we add up the net energy for all of this process, all we need to do is add negative 589 to plus 145. So what we end up getting is the net energy change is going to be negative 444 kilojoules per mole, so you can see that, in fact, it is very favorable for neutral sodium and neutral chloride to form sodium chloride in an ionic bond. And the net increase then, is a decrease in energy.
So, I just gave you the number in terms of what that Coulomb potential would be in attraction, but we can I easily calculate it as well using this equation here where the energy is equal to the charge on each of the ions, and this is just multiplied by the value of charge for an electron divided by 4 pi epsilon nought times r, are r is just the distance in terms of the bond length we could talk about.
So, let's calculate and make sure that I didn't tell you a false number here. Let's say we do the calculation with the bond length that we've looked up, which is 2 . 3 6 angstroms for the bond length between sodium and chloride. So we should be able to figure out the Coulombic attraction for this.
So, if we talk about the energy of attraction, we need to multiply plus 1, that's the charge on the sodium, times minus 1, the charge on the chlorine, times the charge in an electron, 1 . 6 0 2 times 10 the negative 19 Coulombs, and that's all divided by 4 pi, and then I've written out epsilon nought in your notes, so I won't write it on the board. And then r, so r is going to be 2 . 3 6 and times -- what is angstrom, everyone? Yup, 10 to the negative 10. So 10 to the negative 10 meters. So, if we do this calculation here, what we end up with is negative 9.774 times 10 to the negative 19 joules.
So that's what we have in terms of our energy. That does not look the same as what we saw -- yup, do you have a question?
STUDENT: [INAUDIBLE]
PROFESSOR: OK. Luckily, although, I did not write it in my own notes, I did it when I put in my calculator, thank you. So you need to square this value here and then you should get this value right here, negative 9.77. All right, so what we need to do though is convert from joules into kilojoules per mole, because that's what we were using. So if we multiply that number there by kilojoules per mole -- or excuse me, first kilojoules per joule, so we have 1,000 joules in every kilojoule. And then we multiply that by Avagadro's number, 6.022 times 10 to the 23 per mole. What we end up with is negative 589 kilojoules per mole. So this is that same Coulombic attraction that we saw in the first place.
So, notice that you will naturally get out a negative charge here, remember negative means an attractive force in this case, because you have the plus and the minus 1 in here. So we should be able to easily do that calculation, and what we end up getting matches up with what I just told you, luckily, and thank you for catching the square, that's an important part in getting the right answer. So, experimentally then, what we find is that the change in energy for this reaction is negative 444 kilojoules per mole.
If we look experimentally what we see, it's actually a little bit different, it's negative 411 kilojoules per mole. So, in terms of this class, this is the method that we're going to use, and we're going to say this gets us close enough such that we can make comparisons and have a meaningful conversations about different types of ionic bonds and the attraction between them.
But let's think about where this discrepancy comes from, and before I do that I want to point out, one term we use a lot is change in energy for a reaction where, for example, you break a bond. Remember that the negative of the change in energy is what's called delta e sub d. We first saw this when we first introduced the idea of covalent bonds. Do you remember what this term here means, delta e sub d? A little bit and some no's, which this was pre-exam, I understand, you still need to review those notes, it's dissociation energy. So you get a negative energy out by breaking the bond. The dissociation energy means how much energy that bond is worth in terms of strength, so it's the opposite of the energy you get out of breaking the bond -- or excuse me, the energy that you get out of forming the bond. It's the amount of energy you need to put in to break the bond is dissociation energy. It takes this much energy to dissociate your bond, excuse me.
All right. So, let's take a look here at our predictions, so I just put them both ways so we don't get confused. The dissociation energy is 444. The change in energy for forming the bond is negative 444. We made the following approximations, which explain why, in fact, we got a different experimental energy, if we look at that.
The first thing is that we ignored any repulsive interactions. If you think about salt, it's not just two single atoms that you are talking about. It's actually in a whole network or whole lattice of other molecules, so you actually have some other chlorines around that are going to be having repulsive interactions with our chlorine that we're talking about. We're going to ignore those, make the approximation that those don't matter, at this point, in these calculations. And the result for that is that we end up with a larger dissociation energy than the experimental value. That's because the bond is going to be a little bit more broken than it was in our calculation, because we do have these repulsive interactions.
The other thing that we did is that we treated both sodium and the chlorine as point charges. And this is what actually allowed us to make this calculation and calculate the Coulomb potential so easily, we just treated them as if they're point charges. We're ignoring quantum mechanics in this -- this is sort of the class where we ignore quantum mechanics, we ignored it for Lewis structures, we're ignoring it here. We will be back to paying a lot of attention to quantum mechanics in lecture 14 when we talk about MO theory, but for now, these are approximations, these are models where we don't take it into consideration. And I think you'll agree that we come reasonably close such that we'll be able to make comparisons between different kinds of ionic bonds.
All right. So, the last thing I want to introduce today is talking about polar covalent bonds. We've now covered the two extremes. One extreme is complete total electron sharing -- if we have a perfectly covalent bond, we have perfect sharing. The other is electron transfer in terms of ionic bonds. So when we talk about a polar covalent bond, what we're now talking about is an unequal sharing of electrons between two atoms.
So, this is essentially something we've seen before, we just never formally talked about what we would call it. This is any time you have a bond forming between two non-metals that have different electronegativities, so, for example, hydrogen choride, h c l. The electronegativity for hydrogen is 2.2, for chlorine it's 3.2. And in general, what we say is we consider a difference in terms of a first approximation if the difference in electronegativity is more than 0. 5, so this is on the Pauling electronegativity scale. So what we end up having is we sort of have a kind of, and what we call it is a partial negative charge on the chlorine, and a partial positive charge in the hydrogen. The reason we have that is because the chlorine's more electronegative, it wants to pull more of that shared electron density to itself. If it has more electron density, it's going to have a little bit of a negative charge and the hydrogen's going to be left with a little bit of a positive charge.
So, we can compare this, for example to, molecular hydrogen where they're going to have that complete sharing, so there's not going to be a delta plus or a delta minus, delta is going to be equal to zero on each of the atoms. They are completely sharing their electrons.
And we can also explain this in another way by talking about a dipole moment where we have a charged distribution that results in this dipole, this electric dipole. And we talk about this using the term mu, which is a measurement of what the dipole is. A dipole is always written in terms of writing an arrow from the positive charge to the negative charge. In chemistry, we are always incredibly interested in what the electrons are doing, so we tend to pay attention to them in terms of arrows. Oh, the electrons are going over to the chlorine, so we're going to draw our arrow toward the chlorine atom.
So, we measure this here, so mu is equal to q times r, the distance between the two. And q, that charge is just equal to the partial negative or the partial positive times the charge on the electron. So this is measured in Coulomb meters, you won't ever see a measurement of electronegativity in Coulomb meters -- we tend to talk about it in terms of debye or 1 d, or sometimes there's no units at all, so the d is just assumed, and it's because 1 debye is just equal to this very tiny number of Coulomb meters and it's a lot easier to work with debye's here.
So, when we talk about polar molecules, we can actually extend our idea of talking about polar bonds to talking about polar molecules. So, actually let's start with that on Monday. So everyone have a great weekend.
Free Downloads
Free Streaming
Video
- iTunes U (MP4 - 111MB)
- Internet Archive (MP4 - 111MB)
Caption
- English-US (SRT)