Flash and JavaScript are required for this feature.
Download the video from iTunes U or the Internet Archive.
Topics covered: Polar covalent bonds; VSEPR theory
Instructor: Catherine Drennan, Elizabeth Vogel Taylor
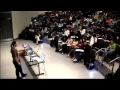
Lecture 13: Polar Covalent ...
Related Resources
Lecture Notes (PDF - 5.1MB)
The following content is provided under a Creative Commons license. Your support will help MIT OpenCourseWare continue to offer high quality educational resources for free. To make a donation or view additional materials from hundreds of MIT courses, visit MIT OpenCourseWare at ocw.mit.edu.
PROFESSOR: -- 10 seconds to answer the first clicker question. As you can see we're having another competition today, so see if you can beat out Justin's recitation and get the most correct today.
So, this is a topic from Friday, which is asking us which of the following molecules are free radical species. OK. So it looks like most and you got it, that it's o h. Let's quickly go over why that is just in case you weren't one of that 74%. So, if we're looking at o h versus c o, what are we looking at to decide if we have a radical here? Valence electrons. So we have 6 plus 1 in terms of o h, that's 7, versus having 4 plus 6, which is 10. Specifically, what do we look for in terms of valence electrons? Odd numbers. So, we have a radical here with o h, whereas we have an even number of valence electrons for c o, so that's not a radical, in terms of what we know how to think about so far, which is using our Lewis structures to determine that.
All right. So that's a little review from Friday, let's move on to today's lecture. In terms of what we're going to be talking about today, we're going to finish up with talking about polar covalent bonds, and finish up with discussing the idea of polar molecules. And then we'll move on to start talking about well, we know how to draw our Lewis structures, how can we use those Lewis structures to think about the actual shapes of molecules.
So, first thinking about our polar covalent bonds. What we had established is if we have a case where we have a covalent bond, but there's unequal sharing of electrons between the two atoms, namely because they have different electronegativities, what we see is we have a polar bond or a polar covalent bond where one of the atoms is going to pull away more of the electron density from that bond, giving it that partial negative charge where the other atom is going to end up with a partial positive charge.
In terms of thinking about well, what do we call a covalent bond versus a polar covalent bond versus a purely ionic bond, it turns out that the distinction is a little bit fuzzy, it's not a clear line, but in terms of talking about things in general, and especially in thinking about problems for this course, what we're going to say is if you have an electronegativity or chi difference between the two atoms that is greater than 0 . 4, and less than 1 . 7, and we're talking about the Pauling electronegativity scale here, which is what's used throughout your book. If you have a difference in electronegativity anywhere in that range, we'll have what we call a polar covalent bond between those two atoms.
So, for example, if we compare the chi values for hydrogen versus carbon, we see they only have a difference of 0.4 . 0.4 is not greater than 0.4 . So we would not call this a c h bond, we would not say it's polar covalent, we would just call it covalent. In contrast, if we talk about a carbon oxygen bond, now we have a chi difference of 0.8 , so that is greater than 0.4 , so we'll call carbon oxygen bond polar covalent.
We can extend this idea of talking about these polar covalent bonds to thinking about an entire molecule. I'm sure you've all heard of the term a polar molecule versus a non-polar molecule, and essentially, when we talk about having a polar molecule, what we're saying is that there's is a net non-zero dipole moment within the whole molecule. So essentially, what we have to do is combine all of the individual bonds to think about the molecule as a whole.
So, let's take an example of carbon dioxide here, or c o 2. Very soon in the lecture you'll learn how to predict shapes based on Lewis structures. So you will quickly see that this is a linear molecule. We can think about the 2 bonds within it. There's two carbon oxygen bonds, and we know that there is a dipole in that bond, which is going toward the oxygen. Remember, in chemistry we always draw the arrow, we're always interested in what those electrons are doing. So if we draw in the bond dipoles, and you can draw these into your notes, you want to draw an arrow towards the oxygen in each case.
But we're not just interested here in thinking about do we have a polar bond, we actually want to know in general do we have a polar molecule. So, looking at c o 2 as a whole, do you think we have a polar or a non-polar molecule here? Non-polar. OK, very good for all of you that said non-polar. The reason it's non-polar is we simply have two equal vectors in opposite directions, so they cancel each other out. The net effect is that we have a 0 dipole moment in the c o molecule.
In contrast, we can look at h 2 o. H 2 o actually has this bent shape, and again, we'll see very soon and how to predict that h 2 o has a bent shape, that water is bent. And again, we do have dipole moments -- we have bonds where there is a polar bond and we should point our arrow toward the oxygen, because electron density is being pulled from the hydrogen atoms to the oxygen. And again, we can cancel some of these vectors out, but we still have a net dipole moment that is going to be going up if we think about combining these 2 vectors here. So, what we would say, which is what we know from everything we've always heard, which is that water is a polar molecule.
So, this is a great way to think about molecules in general. It's very easy to think about if those vectors cancel each other out or if they're additive when we're talking about just single bonds, so we have two atoms or if we have just three atoms, as we do in these cases here. But it turns out, a lot of the molecules we consider are actually much, much, much larger than just a couple of atoms.
So we can't often think about canceling all the different vectors out. And in general, when we're talking about these really large molecules, whether they're large organic molecules or we're talking about proteins, which can have thousands of atoms in them, instead of adding up all those individual polar bonds, what we do is we talk about the number of polar groups that it will have in the molecule. So we can think about a protein, for example, as having a lot of different polar groups or one that has not too many polar side groups. That's one way you hear people talking about proteins, and that has a lot to do with their solubility in water, and also about how the protein's going to fold.
But let's look at a little bit about less complicated example than a protein with thousands of different atoms. Let's instead consider a couple of vitamins here, which instead have a few dozen different atoms in them. Specifically, let's look at vitamin A and vitamin B9. Does anyone know another name for vitamin B9? I don't think I hear it -- does anyone say folic acid. It's also sometimes called vitamin M. It's one of the vitamin B9 vitamins, there's actually other forms of vitamin B9, but this is one of the big ones that you hear about. Hopefully you're all very familiar with folic acid, because it's an important vitamin to take, especially if you're a woman, especially if there's any chance you ever might become pregnant in any kind of near future time, by accident or on purpose.
And the reason for this is because folic acid deficiency in pregnant women leads to neural tube defects in babies, and your brain develops very, very, very early in pregnancy, the brain of an embryo. So it turns out that a lot of women don't realize they're pregnant while the fetus' brain is developing, and if these women are not getting enough folic acid, you can end up with spina bifida, which is an absolutely devastating and very preventable disease or other neural tube defects. So hopefully, if you're not familiar with folic acid, you'll become familiar at least within the next 10 or 15 years or so before you're thinking about maybe, on purpose, becoming pregnant at some time.
Vitamin A you probably all heard about growing up, getting enough of your carrots so you can see at night. Vitamin A is important for eye health. But just looking at the structure of these two molecules, knowing what we know so far about general chemistry, we can already say a lot about how these are going to function the body or how they'll be treated in the body. And specifically, let's take a look at which of these two molecules has more polar bonds and see what that tells us.
So take a look at your two structures and tell me which has, between folic acid and vitamin A, more polar groups within the vitamin. And this should go pretty quickly since you don't need to have an exact number, we're just looking and glancing and seeing which has more. So let's take 10 seconds on this.
All right, great. So most you said folic acid or vitamin B9, so let's switch back to our notes and take a look at why. So vitamin B9 has more polar bonds. It's very easy to see, once I highlight, that it's not even a close call at all. We have a bunch of different polar bonds in B9 versus just one in vitamin A. Remember, the c h bond we're not calling polar covalent because it only has an electronegativity difference of 0.4 between the two atoms.
So what we know is that vitamin B9, folic acid, is more polar. Remember, we also just said that water is polar, so would we say that B9 is water or fat soluble? It's going to be water soluble -- everyone knows the saying, "like dissolves like." B9 is going to be very water soluble. This actually is very important if you think about taking your vitamins. This means if it's water soluble, any vitamins that are water soluble, and now you should to be able to look at the structure of any vitamin and tell me, for example, that vitamin C is water soluble, that folic acid is water soluble. If you take these vitamins, what happens is that they're very quickly and easily excreted into your urine.
So, for example, some people like to take mega doses of vitamin C. What really happens is that you have a mega dose of vitamin C in your urine, it doesn't stick around long in your body. So it doesn't help and just take all of your vitamin C at once. That's why it's important to eat balanced meals throughout the day, you need to be getting a constant supply of these water soluble vitamins. The same is for folic acid, you can't just take it once a month, you need to be taking it regularly in order that you keep the stores up in your body, otherwise you're going to excrete it, you're going to get rid of it very quickly.
In contrast, if we think about vitamin A, is this going to be water soluble or fat soluble? Yup, so this is the fat soluble vitamin. Vitamin E is another big one that's fat soluble that gets a lot of press in terms of being an important vitamin to take.
We can think about what it means when a vitamin is fat soluble instead of water soluble. Well, now it's not going to get excreted out of our body so quickly, so we actually can build up amounts of vitamin A vitamin E, for example, but that can also pose a problem if you think about biologically what's happening.
And up here I just show two different supplements that I found on the internet. This is One A Day vitamin, it has about 100% of what you need of everything. And then this vitamin is one that I found that's supposed to really help you with your eye health, if you have bad vision instead of glasses, they suggest that you try this vitamin here. And what you can see is that it has five times your daily value of vitamin A, and 13 times what you need of vitamin E. Is this a good idea? No. Basically, you are just building up more and more and more of these fat soluble vitamins into your body. And there have been studies that have come out recently trying to look at the health benefits of vitamin E, and in some of these studies they give these mega doses, and instead what they find is increased bleeding in these patients, an increase in overall different types of death that can be happening.
You want to take your vitamins, it's very important, but you don't want it just build up, and you want to think about is the vitamin that I'm going and getting 800 times what I need a day, is that a water soluble vitamin or a fat soluble vitamin, and using your chemistry, you should be able to very quickly take a quick look at that structure and figure out what kind of a vitamin that is.
All right. So that's just one reason we would want to be able to think about polarity is thinking about whether something is very water soluble or not. Let's think about some other things that have to do with polarity and then can tell us a lot of other information. So let's move on to talking about the shapes of molecules. And the shapes of molecules is very important for a number of different properties when we're thinking about chemical reactions and reactions that take place in the body. When we talk about shapes of molecules, we're talking about the geometry of that molecule. And the geometry influences all of its different properties, including things like melting point or boiling point, it's reactivity.
We just saw when talking about polar molecules, that it influences whether or not the molecule itself is polar or apolar. It's also really important when we talk about biology. Shape is particularly important when you think about enzymes having an active site where a molecule needs to fit perfectly into the active site, it does that because of its shape.
A quick example that we can think about is with sucrose. Does anyone know what sucrose is? It's just table sugar -- sucrose is that crystal in sugar that we hopefully use in most of our sugar intake as sweeteners. A lot of times we use corn syrup, which is often not sucrose and which tends to be not always as good for us, although anything in too much excess is obviously a non-ideal situation.
But in order for us to use the energy from sucrose, sucrose is made up of two individual sugar monomers. It's made up of a monomer of glucose and one of fructose, a 6 and a 5 membered ring, so in order for our body to use it, we need to break it into with its monomers. And if we that, we can do it by what's called hydrolysis -- sucrose and water breaks down into its two monomers, now our body can use it. The problem is this process takes on the order of 10 or maybe 100 years in order for us to get enough of the sugar broken down.
That's why we need an enzyme molecule, and the enzyme in our body is called sucrase. Sucrase breaks down sucrose, it catalyzes that reaction, so it happens very quickly. And the key is, and here's a ball and stick shape of what sucrose looks like. It needs to fit exactly into that active site. When it does, it combines into the enzyme, the enzyme can then catalyze the hydrolysis or the breaking apart of those two individual monomers into its separate pieces. And then it let's go of the glucose and the fructose, and we get our enzyme back again, and something else combined into that active site.
So, this is one very simple example of why molecular shape is very important. A lot of times you're thinking about small molecules interacting with proteins or interacting with other molecules, and you want to think about the shape of that molecule to think about how that interaction is going to take place.
So what we're going to use to think about molecular shape or molecular geometry is what's called valence shell electron repulsion or vsper theory. And this theory is very convenient for us to be thinking about because we are now masters of drawing Lewis structures, and vesper theory is based on Lewis structures, and also the principle that when we have valence electron pairs, they're going to repel each other. This make sense any time you have negatively-charged electrons, you want to get them as far away from each other as possible because they're all negatively charged.
And the other principle is that the geometry around that central atom, which you've identified as the central atom in the Lewis structure, is going to be such that it minimizes the repulsion between those either bonding electrons or the electron pairs. So when we talk about vsper there's a special nomenclature that we do use, and in vsper we have a -- does anyone know what a means in vsper theory? It's a central atom. What about x? So this is actually the bonding, any bonding atom you call x. And then we have e, which is equal to lone pairs. So e is a lone pair -- e is not a lone pair electron, so if you have two electrons, that's one lone pair.
All right. So there are some guidelines that we use when we're coming up with these vsper geometries. The first thing we talk about is the steric number, which we use to predict what that geometry will be. When we're talking about steric number, all we're talking about is adding together the number we have of bonded atoms, plus the number of lone pairs. So, essentially were just adding x to e and that gives us our steric number.
So, for example, if we look at this molecule, which is a x 2 e, what is the steric number here? Yeah, it's 3, so we have a steric number of 3. Something that I want to point out is that we could have a different molecule, which is also a x 2 e, but in this case we have a double bond between the central atom and one of the x atoms here, and what I want to point out is in vsper theory we treat bonds as bonds, we don't worry about if they're single or they're double or they're triple. So again, we call this a x 2 e, this again, has a steric number of 3. So what's important in terms of vsper theory is the number of atoms bonded to a central atom. What's not important is the types of bonds that we're dealing with.
A few other guidelines I want to mention is first of all, to think about resonance structures. So on Friday we were talking about the chromate anion, and we said that here are two of its resonance structures here, but that we could actually draw four more resonance structures, and I just want to point out when you take a molecule that has many different resonance structures and you want to draw its vsper, it's Lewis structure and its vsper geometry, you can take any single one of those resonance structures, it doesn't matter, you'll all end up with the same geometry.
And lastly, if we're talking about a molecule that has more than one central atom, which is what we're very, very often doing, you need to deal with each one separately. So for example, with methanol here, we have a carbon, which is a central atom, and we also have an oxygen, which is a central atom, you need to talk about the geometry separately, we don't talk about the geometry of the entire molecule.
So, let's go ahead and look at some of these vsper examples, and Professor Drennan is going to help demonstrate what some of these are with some models here.
So the first case we're going to talk about is without any lone pairs -- this is the most straightforward case. And our first case that we can have is a x 3, which is going to have a linear shape, and that will have a bond angle of a 180 degrees.
The next case that we can talk about is trigonal planar or a x 3. Now, you do need to know these geometry, you need to know the names. A lot of them are very easy to remember. I expect not too many of you will get linear incorrect. Also, trigonal planar, pretty easy. Trigonal, it has 3 atoms around the central atom and the molecule is planar.
PROFESSOR: So, what is the bond angle for this geometry? 120.
PROFESSOR: All right. Great. So, next we can think about a x 4, and this is what's called a tetrahedral geometry. If you're trying to remember this name you can think of each of those bonding atoms as being in the corner of tetrahedron. One thing I want to point out is you're seeing some notation we haven't used before in this class where you have a wedge coming out at you, and you also have a dashed line to one of those bonds. Any time you see a wedge in a Lewis structure, it means that it's coming out at you -- it's either coming out of the screen or it's coming out of your paper. And any time you see a dashed line here, which might be easier to see in your notes, that means that the bond is actually going into the page or into the screen or into the blackboard.
PROFESSOR: And what are the angles for a tetrahedral?
PROFESSOR: All right, so they're 1 0 9 . 5. Pretty close. So that's as far away as those bonds can get from each other would be 109 . 5 degrees.
The next case we have is a x 5. That is what we call trigonal bipyramidal. Again, we have trigonal, because we have those 3 bonds in the center, and if you can picture putting walls between all those bonds, you can see there's a pyramid on the top and a pyramid on the bottom, so bipyramidal.
PROFESSOR: And there are 2 sets of angles here, what are the angles for equatorial atoms? 120. And then for axial? 90.
PROFESSOR: And again, axial, those are just the ones in the axis, and equatorial if you put your globe around the equator.
So the last case we have is a x 6. A x 6 is what we call octahedral -- you can picture each of those bonded atoms as a corner of an octahedron.
PROFESSOR: And what are the angles in this, this one set of angles? 90.
PROFESSOR: Great. So, 90 degrees.
All right. So this is all straightforward when we're just thinking about different molecular shapes that don't have any lone pairs in them, but once we start having lone pairs, we now need to think about how those lone pairs are going to affect the geometry. But before we do that, let's talk about some examples of molecules without lone pairs.
So the first case is what we saw at the beginning of class, carbon dioxide, and we said that that was linear, and now we know why it's linear -- it has a formula of a x 2, and an s n number of 2. And that's a bond angle of 180 degrees.
So, you can tell us about borane, borane is a x 3.
PROFESSOR: And what is the geometry? And the angle? 120. Feel free to yell out very loudly, we want people in OpenCourseWare to hear the answers coming from the room.
PROFESSOR: All right. So the next case we're going to look at is c h 4 or methane, and let's do a clicker question here to make sure everyone is remembering these geometries. And this should be very quick, and try to not turn the page back one, and tell us in 10 seconds here what the geometry is.
98%, that is a new record. Very good. Tetrahedral.
PROFESSOR: All right, while I'm holding carbon dioxide in one hand and methane in the other hand, I just want to do an additional plug for the energy debate tonight. So there will be representatives from both presidential campaigns that are going to be there to answer questions. And I'm not sure of the exact format, I'm not sure whether all audience members are going to be able to ask questions or not. I'm not sure if I'm going to be there, I'm supposed to be giving a talk actually, ironically, about energy at the same time. But if I can't make it and you're allowed to ask questions, I'd like you to ask a question for me.
So, my lab looks at enzymes that remove carbon monoxide and carbon dioxide from the environment. And I have an energy initiative grant to do this, because MIT recognizes that an important part of any energy initiative is thinking about lowering pollutants and greenhouse gases.
So, I heard on Thursday at the debate when Governor Sarah Palin was asked directly if she really doesn't think there's any connection between global warming and any man-made activities, that she said, well, you know, it could be just regular temperature fluctuations, and she doesn't want to point any fingers. So, she's not quite sure. I also learned on Thursday that she will head the energy initiative in a McCain/Palin White House.
So, if you go to this debate, I would like you to ask what is your plan for sequestering carbon dioxide and other greenhouse gases. You can ask both candidates, and please let me know what the answer is, because I think that's a really important question -- here we have carbon dioxide, here we have methane, we need to be thinking about greenhouse gases.
PROFESSOR: You can also throw in the question that you do know this is tetrahedral and 109 . 5 degrees -- show your chemistry knowledge here.
All right, and let's keep showing that we know our geometries, let's look at p c l 5.
PROFESSOR: And what is geometry here? Yup. And the angles? 120 and 90.
PROFESSOR: So, 120 equatorial, and 90 axial. And last we have s f 6.
PROFESSOR: So, what is the geometry here? Octahedral. And angle? 90.
PROFESSOR: All right, so there's our set of examples, one for each for those shapes or geometries that have no lone pairs. And now let's think a little bit about once we do have molecules with lone pairs. And the biggest point to keep in mind when we're comparing lone pair electrons with bonding electrons or electrons in bonds, is that when you have electrons in bonds they have less spatial distribution than lone pairs. So that's just a way of saying that electrons and bonds, they take up less space.
So, when we think about lone pair electrons, they're taking up more space and this means that they're going to experience more repulsion in thinking about the lone pair electrons with either other lone pair electrons or with bonding electrons. So let's think a second about the order that this will be in. The biggest repulsion we would feel is if we have two different lone pair electrons or lone pairs. They're going to have the most repulsion. In the middle is if we're talking about a lone pair with a bonding pair. And then the least repulsion is going to be between two bonds or two bonding pairs of electrons.
So, let's look at an example of where this comes up. So the first example I'm going to talk about is a molecule that has the geometry of a seesaw shape, and once we get to having Professor Drennan actually show you that shape, you will never forget. See-saw, that's going to be one of the easy geometries to remember. But the first thing that we need to consider in terms of the shape, which starts, if you picture first of all, and these models sometimes don't quite stay together, we could actually have two different possibilities.
The first is the idea that we could have an axial lone pair, and the second is the possibility that we could have an equatorial lone pair. And we could consider both, and we should be able to use our vsper principles to think about which one is actually going to happen.
So if we think about having an axial lone pair here, that would mean that these lone pair electrons are going to be within 90 degrees of three different loan pairs, and actually we'll see later, it will be more than 90 because they're actually going to push them down. But in terms of considering how many bonding electron pairs they'll repel strongly, what we care about is anything within 90 degrees initially. So, what we would see with the axial lone pair is that we have three lone pairs that we're going to very strongly repel.
Now would you tell me, if we think about an equatorial lone pair, how many different bonding electrons or a bonding pairs that will strongly repel if we have an equatorial lone pair? So you might need to look at your notes to actually compare these two before you submit your answer up here.
All right. Let's take 10 seconds on that. OK, strong showing with the clicker questions today. It's going to be a tight race for clicker competition. It's correct that the equatorial is, in fact, has two, only two that it strongly repels. So, actually it's probably easier to look at Professor Drennan's model here to see that. So it's where the equatorial lone pair is that is the actual seesaw geometry.
PROFESSOR: So, how many of you had seesaws in playgrounds when you were growing up? Oh, a lot of people. I know that they're not considered totally safe anymore, so some of them are going away. So this is seesaw. Now you should never forget it.
PROFESSOR: All right, so seesaw, we've got seesaw. Just to point out a few more shapes. We took one out, we replaced one bond with a lone pair in terms of seesaw. If we have a x 3 e 2 now we have two equatorial lone pairs. This is called the T-shaped
PROFESSOR: T-shaped.
PROFESSOR: See, these aren't too hard to remember the geometries, the geometry names. And we also can think about if we have a x 4 e 2. So now in order to get our lone pairs as far away from each other as possible, they're going to be on the axial position, and this is called square planer.
PROFESSOR: So, this is easy to remember, square, also planar.
PROFESSOR: All right. So, let's talk a little bit now about what the actual angles are between bonds when now we have these lone pairs present. And remember, what we said is when we have a lone pair of electrons, they actually are going to have more repulsion than if we just have a c h bond or a bonded pair of a electrons. So essentially what that means is that in molecules that have lone pair electrons, so for example, if we look at n h 3 or ammonia versus methane here, and Professor Drennan is showing those models to you here. What you end up seeing is actually that the bonding angle in n h 3 between the n h bonds is smaller. And you can't see it with these models because these are not actual lone pairs. But if they were actually repelling each other, they would be pushing those bonds as far away as possible, and instead of being 109 . 5, you'd see that the angle is now 106 . 7. So it's a smaller angle between bonds, because you have more repulsion from those lone pairs pushing those bonds down.
We can also think about the influence of atomic size in terms of this effect. So first of all, what happens to atomic size as you go down the periodic table? Good, it increases. So we have size increasing as we go down the periodic table. Phosphorous is right underneath nitrogen on the periodic table, so phosphorous is going to be bigger than nitrogen. In terms of picturing what happens with those lone pairs, when we have a larger atom, the orbitals are also going to be larger, they can take up more space. That means these electrons, this lone pair electrons are going to take up more space. This means they're going to push away those bonding electrons even more. So would you expect the bond to be larger or smaller for p h 3?
STUDENT: [INAUDIBLE]
PROFESSOR: It's going to be smaller.
So, what we see is that angles between bonded atoms actually decrease as you go down a column on the periodic table. So the actual angles for p h 3 are now going to be really quite a bit smaller, they're 93 . 3 degrees.
All right. So let's create a list for ourselves in terms of all the geometries that we can have now that we're dealing with lone pairs. One thing I want to point out in terms of remembering the names of the different geometries, when you're naming a geometry, the geometry name, for example, when we looked at square planar, we're only actually naming where the bonds are, where actual atoms are. The name doesn't really depend on the lone pairs. And also, when you draw a geometry, you don't always have to draw the lone pairs in, but you have to remember that the lone pairs are very much affecting the angles within your molecule and also the actual shape.
So, for example, let's start talking about different types that have lone pairs in it. First of all, thinking about a x 2 e, so we have one lone pair, and the geometry here is bent, and I want you, thinking about lone pair repulsion, to tell us what you think the angle between these bonds are going to be? And let's take 10 seconds on that.
OK, good. 75%, that's not bad. Let's think about why. It's going to be less than 120 degrees, because we know that normally in a trigonal planar situation, we have an angle of 120 degrees. And since lone pairs are going to cause more repulsion, we're actually pushing down these two bonds here closer together, so what we end up seeing is that they're going to be less than 120 degrees. And it depends on the actual molecule what the exact angles are going to be, so you never have to learn the exact angles in terms of lone pair electrons, you just need to be able to tell us if the bond angle is going to be less than 120 degrees.
So let's look at another example here, a x 3 with one lone pair, e. This is called trigonal pyramidal. Again, you have trigonal because there's three atoms bonded to the central atom, and this looks like a pyramid here.
PROFESSOR: So, what would the angle of this be?
STUDENT: [INAUDIBLE]
PROFESSOR: I think I heard it.
PROFESSOR: I think so, too. Less than 109 . 5. Let's take another example, a x 2 e 2. This is also bent. Tell us what the geometry -- we told you the geometry, tell us what the bond angle is going to be between these bonds. So, let's take 10 seconds on this. Let's re-poll F 4. So 10 seconds again.
All right. In about 10 seconds, Darcy, in 10 seconds we'll hit the next side.
OK, so it's less than 109 . 5 degrees now. So some of you wrote that it was less than 120 degrees, and we can think about if we switch back to the class notes the difference here. So even though, so this is now going to be less than 109 . 5, if we looked at the case we had bent in the first case, that started with 120 and one of those bonds was replaced with a lone pair, but now we have two lone pairs here, so now what we're going to see is that the two bents are not, in fact, equal. The bent where you started with the tetrahedral shape is actually going to be less than 109 . 5, and Professor Drennan can maybe show us that with the models here.
PROFESSOR: It's a little had to see, actually, between the two, but I think it's easier to see on this, that if you consider the sort of starting place for the two, if you look at the bottom set and you put orbitals, so it also depends on your starting geometry. It can be bent if take off these bonds on the top, but it depends on what the starting geometries were. And so you will go with your starting geometry, if it was 109 . 5, it'll be less than that. If it's 120, then it's less than that.
PROFESSOR: So, this is a good case of where, even when you have the geometry the same, you need to think about how many lone pairs you're dealing with in your molecule, and think about what would the geometry be if I just pictured those lone pairs in there as bonds and then push them even closer together so that it's less than that actual angle.
OK, let's talk about a x 4 e, and that is what we call the seesaw shape.
PROFESSOR: And so, what difference in angles are you going to have?
STUDENT: [INAUDIBLE]
PROFESSOR: Yup.
PROFESSOR 2: So, two sets again, one is less than 120, and one is going to be less than 90.
So, let's look at a x 3 e 2, you'll notice there's a lot more combinations we can get to once we're talking about lone pairs. This is called T-shaped, and we had briefly discussed that but not mention what the angles would be.
PROFESSOR: So, what angle would you have here? Yup.
PROFESSOR: All right, great. Less than 90 degrees.
So, we'll start a new page here and talk about a x 2 e 3, and we'll let you tell us what this geometry is. Yup, so I hear a lot of linear out there. And you need to keep in mind we give the names based on where the actual bonds are, not where the lone pairs are, even though the lone pairs, of course, affect the structure in the geometry.
PROFESSOR: The angle?
STUDENT: 180.
PROFESSOR: Yup, 180.
PROFESSOR: So, it's exactly 180 here, not less than.
PROFESSOR: So, for a x 5 e, what we have here is called square pyramidal. This is square because of the four square at the bottom of your pyramid, then you can picture the pyramid as you go to the top.
PROFESSOR: And so what angles would you have here? Yup, less than 90.
PROFESSOR: So, less than 90, great. All right, there are more.
So, our next combination that we can think about is if we have four bonded atoms and two lone pairs, a x 4 e 2, that's going to be called square planar.
PROFESSOR: So, again, we have square and it's planar. And so what are your angles here? Yup, exactly 90.
PROFESSOR: So next let's look at a x 3 e 3. This is what we call T-shape, this is also T-shape.
PROFESSOR: And angle here?
PROFESSOR: Yup, I think we heard less than, it's less than 90 degrees. And let's look at a x 2 e 4, and again, you guys can tell us what this geometry is, first of all. Yup, it's linear. So, we have lots of different ways we can get to a linear molecule, some of which have lots of lone pairs, some of which have no lone pairs at all. This is linear and a 180 degrees.
All right. So we'll do just a few examples to show you some actual molecules that have these geometries. We won't go through all of them, because as we saw, there's lots of different combinations we can have once we start getting into lone pairs. The first we'll look at is water here, and water is a good one to look at because we had seen that at the beginning of class here when we were talking about all polar molecules. When we talk about the formula type of water, what when you say the formula type is?
STUDENT: [INAUDIBLE]
PROFESSOR: A x 2 e 2, that's correct. And what is the geometry of water? It's bent.
So what we saw at the beginning of class is water is bent and now you can see why and should be able to predict that yourself. I just want to mention that if you look, and depending on the edition of the book you have, in one edition it's called bent, in the other edition it's called angular. We'll call it bent, but just remember that bent and angular, they're the same geometry.
Let's look at another example, s f 4. And here it is drawn here. What is the formula type for s f 4? I think I heard it, a x 4 e. And the geometry? Seesaw, good. All right, we never get seesaw wrong, that's the easy give-away one.
All right, what about b r f 3? This is the shape here. What is the geometry for b f 3 -- the original geometry, not the new geometry here? What was that?
STUDENT: T.
PROFESSOR: T-shaped, that's right. B f 3 is T-shaped.
All right, so let's look at xenon f 2. That's going to have a formula type of a x 2 e 3, if we actually look at the Lewis structure here. So if you think about what the geometry is, and you should just be able to look at this and see, what would you say the geometry of xenon f 2 is? STUDENT: Linear.
PROFESSOR: Linear, good.
All right. And let's try one more here, which is a x 4 e 2, or a xenon f 4 -- very explosive, but a good example.
STUDENT: Square planar.
PROFESSOR: Square planar, all right, great.
So, in general, when we think about vsper theory, even though it doesn't talk about or tell us anything about the energies of these different shapes, it's very useful in making a first approximation and coming very close to thinking about what the actual shapes of molecules are. So one thing I want to point out in terms of what you're responsible for is you should be able to fill out one of these charts with only seeing the labels up here -- tell us the formula type, tell us the steric number, tell us the geometry, you should be able to draw the Lewis structure, you already know how to do that, and also talk about the angles.
So, as you finish up your problem-set, you can, I think now, get through just about all of it. You can do now this part with the geometry.
So, we'll see you on Wednesday -- we're going to get out a little bit early today.
Free Downloads
Free Streaming
Video
- iTunes U (MP4 - 98MB)
- Internet Archive (MP4 - 99MB)
Caption
- English-US (SRT)