Flash and JavaScript are required for this feature.
Download the video from iTunes U or the Internet Archive.
Topics covered: Genetics 1
Instructors: Prof. Eric Lander
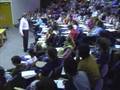
Lecture 6: Genetics 1
I am the other half of the teaching team for 7.01.
You've already gotten to meet my good colleague Bob Weinberg.
My name is Eric Lander. And Bob and I are both faculty here in the Biology Department. In fact, we're both members over at the Whitehead Institute for Biomedical Research.
In fact, we just spent the whole weekend together at the Whitehead Retreat. And so, Bob and I have been doing this course together for a number of years. And we very much love it. I am -- I'll take a brief moment and introduce myself, since I haven't had the opportunity to do so yet. I am by training, well, actually, I'm really a geneticist. By training I'm actually a pure mathematician.
That was actually what my undergraduate degree was in, and even my PhD was in, but then wandered into biology.
And for the last almost 20 years, I have been doing genetics in some form or another. So I love genetics and look forward to talking a lot about genetics. And it's really lovely that my first lecture today is actually going to be our first introduction to genetics. I am -- Just for other backgrounds, I direct this new Broad Institute that is here. And it's actually a joint institute between MIT and Harvard. And you will know it now as a hole in the ground next to Legal Seafood.
If you see a bunch of cranes and things opposite the biology building and opposite Legal Seafood next to the Whitehead, that's the Broad Institute. And we have ambition some day to be more than the hole in the ground but to actually rise above the ground.
And the Broad is about genomic medicine and using genomes and things like that. And the Broad Institute includes this center at MIT that was one of the leading participants in the Human Genome project. So that's a lot of what I do with my day job, in addition to teaching, is work on things like the Human Genome project. And, now that we actually have a sequence to the human genome, figuring out what in the world it all means. And I hope I'll get a chance to tell you, during the course of this class, about the human genome and about what's in it and things like that. Like I say, that's one of the things I tremendously love about teaching biology as opposed, if I can get in trouble, to any of the other required introductory courses, is that our curriculum changes every year because the field is moving so rapidly. I look back at what we taught ten years ago in this course, because I've been teaching it that long, and all sorts of open questions now we know the answers to and are part of the curriculum. Some of the things we thought we knew we now know are false and we know new things.
And every year we get to introduce new stuff.
And I know, I mean with all due respect to calculus, it's just not the case for calculus that there's anything really new to introduce. Most of it sort of settled down about three or four centuries ago. And, you know, that's just not the case with what we do. Anyway, so that's why I love it. All right. So Bob has been talking to you about biochemistry largely. And I'm going to now turn to genetics. But I want you to understand that that is an overarching framework that explains how all the materials you're going to see, at least in the first half or more of this course fit together.
And Bob may have mentioned it, but I'm going to mention it again, I would use this following diagram as kind of our roadmap or subway map of where we're going in this course. What we really want to do is understand biological function. That's what we most want. How is it that an organism is able to breathe in air and distribute it to its cells? How is it that an organism is able to move its muscles?
How is it that an organism is able to fight off invaders to its body, microbes, things like that? How is it that an embryo develops into a full adult? Zillions of questions. That's what I mean by biological function. The two complimentary approaches to studying biological function, over the course of the past century or so in biology, have been the following. There have been the biochemists.
Biochemistry seeks to break down the organism into individual components and study them on their own in a test tube.
They will take an organisms, and to a biochemist wishing to study the beauty of a butterfly flapping in the wind and understanding all of the mechanics of how it could possibly flap those wings and all, he or she would start by taking the butterfly, putting it in the blender, pressing puree and making an extract, and trying to purify individual components that would explain muscles moving back and forth and all that. This is, of course, a geneticist's point of view, but it's all right. You have Bob who will represent biochemistry just fine. And they want to purify out individual components. Individual components away from the organism. And the most important individual type of component that they study are proteins because there are zillions of proteins and they do all sorts of things in the body.
And so you could say, in some sense, that this whole theme of biochemistry, which got started at the turn of the 20th century, really just a few years before the turn of the 20th century, of grinding up an organism, studying its components and being able to find, for example, I want to understand how I can digest lunch. Well. Or how yeast can digest the sugar.
Grind up yeast, fractionate it and find some protein that's able to digest the sugar all by itself without the rest of the organism, an enzyme to do that. That's the logic of biochemistry.
Genetics is the complimentary point of view. Genetics is the study of organisms minus one component. Of course, what I mean by that are mutants. The geneticist who wants to understand the butterflies and how the butterfly can fly would isolate butterfly strains that have lost the ability to fly.
And ideally one is extremely closely related to the normal butterfly, but for some reason, ideally due to the mutation of a single component they're now unable to fly. And the geneticist would then say, ah-ha, that component must matter an awful lot for the ability to fly because the butterfly that lacks that component cannot fly. It's a totally complimentary point of view. And the objects the geneticists study in order to do that are genes. Now, what is of course hard for you guys to understand but will form a structure for some of the lectures that I'm going to give over the continuing part of this course, is that through most of the 20th century the folks who studied biochemistry and tried to understand proteins and the folks who studied genetics and tried to understand mutants had nothing to say to each other. They didn't speak the same language.
They had nothing to relate to each other by because there was no idea of how this gene stuff, which started as a totally abstract business, could possibly relate to this protein stuff which started as a very practical in the test-tube thing. And they went for a very long time as if they were just ships sailing in the dark unaware of each other. And I exaggerate, but it's more true than not.
The great intellectual event was the unification of these two points of view through the discipline of molecular biology.
Molecular biology was the discipline that realized, oh, my goodness, these are two different sides of the same coin. That, in fact, genes encode proteins, proteins are encoded by genes. Ah-ha. This was a wonderful and important thunder clap in the 20th century. Now, it was a theoretical piece of information at first.
The idea that genes and proteins were related in this way was abstract, very important, but you couldn't do anything really with it, because it turned out you couldn't actually work with individual genes. The next great revolution of the 20th century was a technological revolution that let you actually work with genes. And that was the recombinant DNA revolution in which the tools to be able to study genes on their own away from the organism, study proteins, use genes to figure out what protein they encode, given a protein and figure out what the gene is, given a gene and actually go in and make a mutant in it, not wait for a random one to rise in the lab but deliberately knock it out, all of that operationalized this intellectual procedure, this intellectual framework. So that is, in some sense, a roadmap to coming lectures that I'm going to give.
I'm going to talk about genetics, I'm going to talk about molecular biology, and I'm going to talk about recombinant DNA.
That's the structure of the next several weeks of this course.
And what I want you to do is to recognize that although we're going to dive down into the individual components of it, everything we're going to do over the coming weeks fits into this very amazing intellectual framework. And this is the intellectual framework that you inherit as the new students coming into this field and going into the 21st century is all this was worked out in the last century. You now have an understanding of how all these pieces fit together, or at least you will, how these can be used to study biological function and, as I will also talk about, the recombinant DNA has grown into a world of genomics that has given us the complete picture of all of the components. It's actually not bad.
You were very wise to have shown up when you did because an awful lot of that groundwork has now been laid. You know, if you would have come along 50 years earlier, you know, all that would have been slogged through. Right now you have this laid out for you very nicely. And that's sort of what the theme will be. OK? I would ask are there any questions, but there should be a zillion questions about that.
This is just intended as a framework there.
So let's now dive in. Section 1. And I'll give a bit more background today than I will in some of the other lectures, but we've got to get going. What I really want to do first is talk about, in fact, most of today will be about Mendel.
I confess, Mendel is my hero. He is one of my absolute heroes in science. I just love Mendel. And so I'll dwell on him a little bit today. Now, here's the problem with trying to tell you about Mendel. You already know about Mendel, right? Who here hasn't met Mendel and the peas and the stuff and all that in their high school textbooks? So what am I doing talking about Mendel today? Well, I think what you learn about Mendel in the textbooks in high school does not really bring out what really went on with Mendel's thinking, what's really important about those experiments, what's really interesting.
And so I want to ask you to put aside what you think you know about Mendel and let's go back over the setting of who Mendel was, what he was doing, how it all adds up. Because I think in Mendel you can find just the seeds of how to do great science.
Now, for starters let me clear up, I'll take five minutes to clear up, four minutes to clear up some misconceptions about Mendel.
It has generally been written that Mendel was this monk working in this monastery often in the Chez Republic, at that point in the Austro-Hungarian Empire, and he was isolated, working by himself, and it was amazing he discovered all this stuff.
It's nonsense. Mendel working on genetics was no accident.
It was the result of extraordinary historical and economic forces over the course of about three centuries that culminated Mendel.
Let me briefly explain why. It starts with the Age of Exploration. Europe starts sending out boats around the world, explorers to meet other parts of the world in the 1500s.
The boats come back. They bring back stories of amazing lands. They also bring back odd plants, odd animals.
People begin to look at these plants and animals.
They begin to cross them, grow them and cross them, and look at the weird odd combinations of things that are going on.
And they say, wow, there's so much more variation out in the world than we thought about. Some of it's kind of useful. We can make new kinds of varieties of plants different than we had before, new kinds of varieties of apples. Now, it turns out that's not just an intellectual curiosity that that was the case because economics was changing in the face of Europe in the 1600s and in the 1700s with better transportation networks. So if you happen to be able to make a better apple, it was good, not just for your family, but you would be able to project that through lines of distribution to larger markets. It became economically sensible to invest your efforts in producing a better crop because you could sell it to more people because unified markets and transportation systems were developing across Europe. And, therefore, economic forces began to work toward getting a hold on the understanding of how you could do better breeding. Now, this turned out to be particularly important to the folks in Central Europe in the Austro-Hungarian Empire, which was the center of the textile industry. They were particularly concerned, in the late 1700s, about the fact that as the center of the textile industry they had to be concerned about the raw materials like wool that they used. Wool you could get from Central Europe, the Spanish had begun producing by breeding better sheep with better wool. This freaked out the guys in the Austro-Hungarian Empire because they were risking now losing this stuff to the Spanish because of their better sheep.
And they began, around 1800, to say we better start understanding how to do breeding. They put together societies to understand better the science of inheritance and breeding.
By 1820, a society which was not about sheep but about plants, in fact, apples and grapes, the Pomological and Enological Society of Braunau was organized. Braunau being the capital of the Austro-Hungarian Empire. And this society got all the town fathers of Braunau together. In those days it was just fathers, you know. Together in Braunau and started this society to encourage the scientific study of agricultural inheritance. They had this big dinner and they were drinking and things, and the speech is actually written down where the president gets up and says, "Some day the world may be as indebted as it is to Isaac Newton for physics. They may be as indebted to the City of Braunau for its contributions to inheritance." Which is just eerie to read that in 1820 in setting up this society. That was their high hopes for what they would do. In particular, the president of this society, one CF Nap was president of the society as a side job, his main job was he was head of the Augustinian monastery in Braunau. So he began keeping an eye out for bright young math and physic students. Basically, you know, MIT kids coming out of high schools. And he identified a bunch of smart ones and attracted them to the monastery and gave them problems to work on. He particularly was impressed with this relatively poor kid, Gregor Mendel, who had been floundering around with a couple of things, didn't have bright family prospects, and attracted him to the monastery to work on problems of inheritance. So this was no accident. This was a biotech incubator that had been set up in the Austro-Hungarian Empire.
Not of the sort we'd recognize today, but it's just fascinating to realize Mendel was not in a vacuum at all. He knew what he was doing here. He really wanted, for the good of mankind, to understand how to improve inheritance. But why do we celebrate Mendel today?
We celebrate Mendel today because he went about it, lots of people were interested in this problem, right?
You could probably find hundreds of people who tried to do something on this problem. Mendel was different because he went about it as a scientist. He went about it with a rigor and a persistence unlike all of his peers at the time. So let's think about what it was that Mendel did. So, anyway, forgive me for the historical digression, but I think it's interesting.
What did Mendel do? Mendel started by taking peas.
Now, he went off to the market and he got different varieties of peas.
And he brought back all of these varieties of peas and he tried growing them. Now, actually, although I don't have the records, I'm sure he did lots more than peas.
He brought probably lots of stuff and he tried growing it.
And the first order of question he wanted to ask is if I study inheritance, I've got to start with something that has constant properties. This seems obvious to you guys, but it was not at all obvious at the time that the most important thing you could do, if you wanted to understand the transmission of traits and crosses and inheritance and all that, is not to set up any crosses. It was first to set up your experimental system and make sure it was rock solid. He probably devoted years to getting varieties of different plants, and in particular settling on peas, with a property that when he had peas with different traits, like whether or not the pea seed was round or wrinkled, which will be some of our favorite traits here, that when you simply selfed this plant, crossed it to itself and looked at the next generation, it bred true. Hard to emphasize how important that was, but this was careful experimental design.
So many biological projects fail because people don't take the trouble to set up a system that's rock solid. They set up a system that's noisy and you're not really sure you're going to be able to interpret the data, etc. So Mendel did that. Very good.
Always, no matter how long you continued to breed these things, you continued to get round or you continued to get wrinkled.
Now Mendel was ready. He was ready to set up his first controlled cross.
So what he did was he took a round pea and a wrinkled pea and he crossed them together. Now, that's again some serious work.
You first have to go along to one of the peas, cut off its little pollen producing organs so it doesn't self-fertilize because peas will self-fertilize. You've got to cut them off early, make sure it doesn't get its own pollen on it. Then you go over to the other one with a paint brush, you get some pollen and you paint the pollen on the first plant. That's how you set up the cross.
If you screw it up you could have self-fertilization or the wind could carry some pollen from something from somewhere else.
So it had to be done very carefully. He set it up.
And his first big-time observation was? Now, again, I know you know all this, so feel free to chime in. In the next generation all the peas were round. We denote generations with an F. F stands for filial meaning children. We sometimes denote them with a G for generation. Anyway, I tend to use F, and most geneticists tend to use F. The parental generation here is called F0, the first generation is called F1, the second generation F2, etc. So why was this a big deal? This was a huge big deal.
If you took a poll, a CNN Gallup poll of Braunau at that time and you ask voters what do you think would happen if I cross a round pea to a wrinkled pea, what do you think the majority of voters would say?
Well, maybe half and half or maybe all a little wrinkled, you know, a little puckered or something like that.
The notion that one trait would be totally dominant over the other trait was by no means the general thinking. And you know what?
It wasn't even the general case. If you took plants, you guys must know. If you take plants and you cross them, the F1 usually looks like some kind of a mix. It's some kind of a blend between the two. And, of course, that's because you're really looking at situations where you're crossing things in which zillions of different traits are being inherited and it's a hodgepodge. But Mendel had a situation here where he got an absolutely crisp dominance of one trait over the other. And so wrinkled completely disappears, round dominates, wrinkled disappears completely.
Now, next he does another generation.
He goes to the second generation. And here he does this by selfing this plant. That is he simply kind of puts a bag over it and lets its own pollen fertilize itself or he takes a little brush and he brushes its own pollen onto it. And in the next generation his remarkable thing was he saw some rounds and some wrinkles.
What was remarkable about that?
Wrinkled came back. I thought wrinkled was gone.
And it didn't come back in some half-hearted way like a little puckered. It came back fully, totally, every bit as wrinkled as the parental wrinkled. And the rounds were every bit as round. These were discrete traits.
Wrinkled reappeared, and it reappeared with no loss.
No change in the phenotype, no change in the appearance. And that was very important because at the time some of the predominant models were blending of traits. And you would never imagine, if I were to take grape juice and water and blend them together to get some kind of pinkish thing that I would be able to separate that back out into clear water and deep dark grape juice.
But somehow this trait had appeared. Thus, the trait was discrete. Big difference. Big news. This trait could be found still lurking there. It was merely hidden in the first generation. Mendel did one other thing, dear to my heart as someone trained as a mathematician, he counted. When he counted up the rounds and the wrinkles he found what?
Sorry? Three to one round to wrinkled? No, it's not. He found 5, 74 to 1,850. That's what he found.
Now, what do you recognize about that?
Three to one? No, it's not. It's 2.96 to one.
It's not three to one. What's this three to one business?
[LAUGHTER] Why isn't there a famous 2.96 to one rule?
No, no, I'm serious. Mendel did one more thing. He counted.
And then he did something a little bit outrageous.
He intuited. He said although the data do not say three to one, notwithstanding your textbook, I think the data are trying to tell me it's three to one. [LAUGHTER] This is part of science.
I'm sorry? Two sig figs, right. You know, this is actually a big deal because so many people are unwilling to kind of look at their data to say what's the data trying to tell me?
And, of course, there are so many people who are too willing to look at their data and say what's the data trying to tell me? Because you can go off the tracks in both directions.
So Mendel tried some experiments, 3.04 to one, 2.91 to one, etc. And occasionally, yes? No. How could he?
Nobody had done this. He had no textbooks he could consult. So do you think it's possible he experimented with other things that didn't show these properties and said maybe these are lousy traits to work on. I'm getting such good results on wrinkled, let's stay with wrinkled for a while. That is an incredible act of experimental judgment to know that some problems are too complicated, we'll come back to them later. It's not cheating.
You get to say this is an interesting problem, I'm going to work on it. Not only that. I'll tell you, occasionally Mendel did these experiments and he got completely abhorrent results. They didn't match three to one at all. You know what he did? He threw out the data. Do you know why? No, not small sample.
Large numbers. He's sitting there in this garden.
You know, I've actually been to Mendel's monastery.
He's in the garden in Braunau. Remember, he's got to go cut off the little pollen producing organs, he's got to paint the stuff. What if he screws up? What if the wind blows and stuff like that? If an experiment was way off, he had to consider the possibility that he just screwed up because he hadn't gotten to it soon enough and pollen had blown in and had fertilized his plants.
Now, boy, that's a dangerous thing to do, discarding data.
But let's be honest. Sometimes experiments screw up.
And if an experimentalist hasn't got enough judgment to know that sometimes you cannot believe the data you also can go wrong.
So Mendel, who sometimes is accused for cheating for that, it's not at all cheating. What you have to do is say, OK, I've got a problem here. I'm going to redo this experiment a bunch more times. I'm always getting about this three to one thing, but occasionally I get something that's way off there and I feel comfortable saying that's an error. You can go wrong with that, but Mendel exercised very good judgment in excluding that rather than trying to muck this all up by saying occasionally I get something weird. So I know the textbook summarizes this beautiful 3:1 ratio, but so much creativity. First discipline of counting and creativity of interpretation went into all of this. So in the modern world what would Mendel do? In the modern world, upon seeing this three to one result which he, I will note, he saw for a couple of other traits.
Actually, what he did next was he wanted to explain -- This was also part of his brilliance. He made a model, the model of what was going on. Mendel said how can I possibly explain this beautiful observation that for round and wrinkled, and for other traits, I observe an approximately 3:1 ratio in the F0, F1 and F2 generations? Mendel, my heart beats for Mendel. Oh.
A mathematician he is. He says let's make a very simple model. Let's assume that there are two factors of the control inheritance of this trait. I'll call them big R and little R.
The round plants have big R and big R. They have two copies of this factor that controls shape. The wrinkled plant has two copies of the factor that control shape, and the copy of the factor they get is different. So the flavor here is big R, the flavor here is little R.
This has two copies, this has two copies.
And let's assume that this plant transmits one at random of its two factors onto the next generation. It will transmit a big R. Let's assume that this transmits one of the two at random.
It will transmit a little R. And that plant there in the middle will be big R over little R. And what will big R over little R be as an appearance? How does he know that that's going to be round? Sorry? From the result.
He knows because that's what happened. It's not an overwhelming reason. But to make the data work he's got to say, well, then this must be round. OK? So no points for that. He's just fitting the data. Then here, when you self this, the two parental gametes transmit either a big R, so we'll put it over here, big R, big R, little R, little R, they transmit. And the offspring are of that type.
You can either get that. Question there?
Could be. So he had some knowledge.
But, of course, this is his model.
He's entitled to make his model. And you're saying he had good reasons to think in these. So everybody knows Mendel's model, right? So, now, in the modern world, the minute you've got data like this and you've got a model to explain it, what do you do?
Publish. So Mendel, let's put Mendel as a young assistant professor who is all fired up about these results, writes this up for publication in Nature. It's a short thousand word letter to Nature, let's say. And he races it off, he emails it to the offices of Nature in London.
Because he's in Europe, he'll use the London office of Nature saying I have this amazing result, I did these crosses.
Here are the results here. And I have a model that explains the data perfectly. What does Nature do? Sorry? Why does it reject it?
Well, the first thing he does is sends it out to referees, right? The way that scientific publication works is it chooses two or three anonymous referees. It sends the paper out anonymously to those two or three referees for comment saying we've received this interesting paper from this young monk in Austria.
What do you think about it? Give us your opinions? Please write back in two weeks, etc. So you're the referees.
You get Mendel's paper. What do you advise Nature?
Publish or not? No. Why not? It's outrageous.
Why? It's never been heard of. Yeah, that's great.
But, I mean, you sound like a very conservative, you know, you cannot write that. You cannot say it's wrong because it's never been heard of. Yeah? Regenerate.
It would be wonderful if referees could regenerate the result themselves, but it's not practical. For one thing, it takes a long time to grow peas. They might not have those strains of peas. The best test really would be independent replication of this, but unfortunately you cannot get the referee to reproduce each result before accepting the paper. So you have to go on the own internal results of the paper. Has Mendel proved his case for this model? How many people vote Mendel has proved his case for the model?
He's my hero. How many people vote that he hasn't proved the case?
How many people are conscience abstainers? [LAUGHTER] OK. Who says he hasn't proved the case? Why? Exactly.
I mean, great, the model fits the data. He had the data first and he made a model to fit it. Big deal. So you would say?
Yes, he should be able to make a variety of predictions.
That would be a confirmation of a model, at least the beginning of a confirmation of a model is he could make some predictions based on a model. But an ex post facto model to explain the data you already have, of course you're going to have one.
It might be a little whacky, but you always make a model to explain your data. That's not the hard thing. Now give me some predictions.
So, guys, give me some predictions. We write back to Mendel saying we find the author's work to be of interest, it's a provocative and unheard of finding, and it's a fascinating model, but it is just a model. We'd like to see some predictions verified.
So what would they be? Sorry? Color.
Oh, show me more traits. OK. Fine. We want to see more traits. In addition to seeing some more traits, and Mendel actually did have more traits in the paper. I'm just simplifying here. Prove this model. What predictions would you make if this model is correct?
Yes? Keep crossing them. So tell me what you would do.
Please send him instructions here.
OK, so you would like me to cross one of the rounds, an F2 round by a wrinkled. What will happen in the next generation?
How do I do that? I don't have DNA sequencing available or anything, so. [LAUGHTER] See what happens.
So what might happen? What is this round plant here?
What might it be? And what are the probabilities of that?
One-third of the time it will big R, big R. Two-thirds of the time it will be big R, little R. If it is big R, big R then the offspring will all be what? Round. If, on the other hand, it is the case that that's big R, little R then the offspring will all be?
They won't be all anything. They'll be half round, a 1:1 ratio of round to wrinkled. OK? That's an odd prediction that a third of the time the offspring from such crosses will all be round and two-thirds of the time the offspring will be 50/50 round and wrinkled. You wouldn't normally think of that, right? That's the kind of thing that has to be done.
And Mendel, of course, did crosses like that. I simplified here. This is really what Mendel did was demonstrated that all sorts of predictions would be satisfied.
Another prediction that Mendel could make, oops.
Stop, stop, stop, stop. Which should be wrinkled?
Oh, my goodness. Oh, wrinkle that pea. OK. Onward.
Thank you very much, Claudette. That's good.
So he made more and more predictions like this. His predictions, for example, let's just take that F1 pea, round over wrinkled here.
If you cross this back with wrinkled then it's pretty simply because then always, if this is an F1 as opposed to an F2, you're going to get a 50:50 ratio of round to wrinkled.
Moreover, these rounds, if you cross them back, will still give you a 50:50, etc. That's science.
That's the heart of science, is being able to look at data, intuit what the data is trying to tell you, build a model and test a model. All of that is in Mendel. OK? So I know you all know Mendel, but this Mendel really. OK? Now, some definitions.
I need to give you, so Section 2, some definitions.
Because I've been skirting around using some words here.
OK? Number one, the word gene. Gene is one of these factors of inheritance controlling a trait.
Mendel didn't use the word gene. The word gene came along much later.
The variant flavors of a gene, big R and little R, are known as alleles from the Greek word meaning other. These are the alternative forms of a gene.
It can come in the form big R, little R. I might write big A, little A. I might write plus for normal and M for mutant.
There are a lot of different notations geneticists use for that.
The word phenotype means appearance. The plant was round. The peas were round. That's a phenotype. The individual was 7" 7' tall.
That's a phenotype. OK? Those are phenotypes. Genotype means the pair of alleles carried by the individual.
Big R, little R is a genotype.
Big R, big R is a genotype. Little R, little R. Those are genotypes.
An important difference between genotype and phenotype.
Other important words so that we can actually talk to each other.
Homozygous or homozygote. A homozygote is an individual who has a genotype that has two of the same alleles. Two copies of the same allele, the individual is said to be homozygous.
And, alternatively, an individual is said to be heterozygous, heterozygote if they have two alternatives.
A couple of other important definitions.
Dominant.
A phenotype round is said to be dominant over a phenotype wrinkled if what? If the heterozygote shows that phenotype, the heterozygote between pure breeding strains. So phenotype one, pheno one is dominant over phenotype two if the F1 of pure breeding strains shows phenotype one. Similarly, we have the word recessive. Now, I'll mention, and you will then proceed to promptly forget, because all of my colleagues forget, dominant and recessive do not refer to alleles. Big R is not dominant.
Round is dominant. Big R is an allele. Now, you say who cares?
The textbooks get this wrong all the time, it's true.
You won't even find the textbooks use this correctly.
They will tell you big R is dominant.
What if it turned out that big R controlled three different traits?
Maybe roundness. An ability to grow with low salt in the soil.
An ability to bloom in May. Some of those traits might be recessive.
Some of them might be dominant. We know examples of that, where the same allele can control multiple traits, some of which show dominance, some of which show recessiveness.
So real card-carrying geneticists try hard to use the word dominant and recessive to refer to phenotypes, not to alleles or genotypes.
Now, since 80% of the facility in the Biology Department don't use the word with that degree of precision, I don't have high hope that you will either. But I'm going to try to say the words dominant and recessive refer to phenotypes. OK? This is a geneticists' kind of hang-up. We all have our shtick, but this one of mine, is that these really do refer to phenotypes. And it's quite important because otherwise you could get quite bollixed up. And I'll come to a case with sickle cell anemia where you won't be able to describe the sickle cell anemia allele as recessive, dominant or co-dominant.
OK? Good. Those are some definitions. They're worth knowing.
If we get those definitions right the rest of it is pretty easy. All right.
So Mendel publishes this paper in 1865. It's accepted.
It appears not in Nature but in the proceeding the Royal Academy of Braunau and it's published. And what happens?
Nothing. It sinks like a stone. Mendel's paper is totally ignored.
Nobody really pays any attention to it. This paper was sent to many people. Charles Darwin has a copy of Mendel's papers in his files.
But, in those days, the way printing worked, in order to read a book you had to slit the pages open.
Darwin never slit the pages of Mendel's paper, so it's pretty clear he never read the paper, even though it had the answer to much of what he wanted to know about evolution.
No one really read Mendel's paper because it was so far ahead of its time, it just was pretty strange. It had all these concepts. And, anyway, you could always dismiss it with that kiss of death of biology "it's just the model". Right? You can kill things with "it's just the model". People were just not prepared to deal with Mendel. So Mendel, in fact, poor Mendel, maybe he had a good time, I don't think, instead didn't really do much more on this topic of genetics per se.
He became an administrator. Became abbot of the monastery and did other things. Worked on meteorology, etc. And we don't really hear from Mendel again. So what really begins to reignite interest in this is the understanding in the late 1800s of chromosomes. Very briefly, cytologists, people studying cells in the microscope. Cytologists are folks who study cells. They noticed these very funny little structures in cells. They noticed these structures that when you stain then with a dye would stain very funny.
They picked up dye in a certain way. And they noticed that they had this very interesting choreography that when a cell underwent mitosis these funny things would divide down the midline and these little x-shaped structures would go to the two daughter cells like this.
That is these Xs would become single individual pieces. Again, you know about these things.
They had no clue what these were. What is the appropriate scientific procedure when you have no clue what something is? You need to give it a name that somewhat covers up the fact that you have no clue what you're talking about because it sounds much better than just saying they are "these funny things". And so they were referred to as chromosomes, meaning literally colored things. [LAUGHTER] You need to understand these sorts of things. OK? So these chromosomes here, these colored things, for lack of any other knowledge of them, that was the property they could be given. Chromosomes.
Look it up. They executed this very interesting choreography during mitosis. That is cell division. Oh, boy, is that going to be noisy.
Someone should shoot it and put it out of its misery. [LAUGHTER] All right.
But what they then noticed was the following. And we're going to run just a couple of minutes over. I'm going to keep it short. But they noticed that when organisms made sperm and eggs rather than normal cell division, they noticed that these chromosomes, instead of all of them lining up on the midline, lined up in pairs.
And the pairs underwent a series of two divisions.
There was a first division which we call meiosis one in which -- -- one copy of each of these Xs went to each daughter cell. Very different than mitosis where the Xs would be split down the middle. Then a second division occurred, meiosis two. And in that each of the daughter cells now the X is divided.
And they got that. This one looked, for all the world, like mitosis. But instead, at the end of the day instead of ending up with four chromosomes, here we end up with only two chromosomes in each gamete, sperm or eggs. And what happened was from this pair, one member of the pair was selected. Now, this is either producing sperm or eggs. When a sperm like that came together with an egg like that and fertilization occurred, you get back to four chromosomes. You all know this. You learned this in high school. But the important point about this was that people said, ha, things lining up in pairs, one copy of each going to the offspring, then a copy from mom and a copy from dad restoring the pair. Sounds just like what that dead monk was talking about. [LAUGHTER] It was just the reason people really didn't think much of Mendel's paper was because it was so abstract. What were these genes? He didn't point to anything. There was nothing concrete. And folks hate that. By contrast they now began to see things and vaguely remembered that this was just like what Mendel's story was about. And three different groups around the world began to redo this work on crosses and all that.
And wonderfully in 1900 three groups simultaneously published papers about this. Now, Mendel's Law is rediscovered.
Now, the explanation here. How does the cytological observations about meiosis explain Mendel's laws of inheritance of traits?
Very simply. All you have to imagine is that big R is being carried on one of these chromosomes. Little R on the other one.
And then half the offspring had big R, half the offspring had little R.
All of Mendel's laws can be implemented by simply assuming that genes and the alleles of those genes live on these chromosomes.
So it's beautiful, except for one problem. You may remember from your high schools that Mendel also had another law about more than one trait, pairs of traits. Not just that we have this segregations of alleles away for one trait. What was his law about two traits? We'll go over this next time. What was his law about two traits, like round and wrinkled and green and yellow?
That they would be inherited independently of each other.
How would that fit into this model? Different chromosomes. They'd be on different chromosomes. But what if I had three traits?
Eventually, if I had, now, peas actually have seven pairs of chromosomes. So if I study eight traits in peas, two would have to lie on the same chromosomes. So then the chromosome model would contradict independent inheritance. So either Mendel cannot be right with this other law of independent inheritance that you learned about or the Chromosome Theory cannot be right of these living on these physical molecules and getting distributed that way.
Right? So we have a deep problem because either Mendel, my hero, is wrong or this chromosome model is wrong.
And the problem is we don't have enough time to resolve this today, so we're going to have to come back on Wednesday and figure out what happens.
Free Downloads
Free Streaming
Video
- iTunes U (MP4 - 146MB)
- Internet Archive (MP4 - 146MB)
Audio
- iTunes U (MP3 - 12MB)
Caption
- English-US (SRT)