Flash and JavaScript are required for this feature.
Download the video from iTunes U or the Internet Archive.
Topics covered: Immunology 2
Instructors: Prof. Robert A. Weinberg
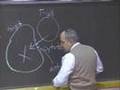
Lecture 23: Immunology 2
Recall, please, that we were discussing last time the fact that the immune system makes a wide diversity of antibody molecules. And, by the way, a synonym for an antibody molecule is an immunoglobulin.
Recall that we used that word very briefly. Another word we use, by the way, was the word antigen. And, an antigen is a functional term.
That was pretty funny. An antigen is a functional term.
An antigen is an agent that provokes an immune response.
And, the term antigen doesn't really refer to any specific chemical structure. It doesn't even have to be a protein. Antigen simply refers to some chemical entity that creates some response from the immune system. We also use the word in passing, epitope. And, an epitope refers to a small region, for example, a protein against which an antibody has developed reactivity. And if you recall our discussion last time, a protein obviously of some size has multiple distinct epitopes, each of which can be recognized by a distinct antibody molecule. And, for us, could we, I'm sorry if I got all this political discussion started, thank you. Thank you. An epitope, from the point of view of a protein is an oligo peptide.
So, a stretch of amino acids of maybe ten, or 12, or 15 amino acids forms an epitope. And therefore, you can imagine there are multiple epitopes on the surface of a protein.
In fact, antibodies can sometimes recognize the internal parts of the protein because protein can sometimes become denatured or degraded. Indeed, as we will discuss shortly, proteins also become cleaved into oligo peptides.
These oligo peptides can come from any part of the protein, to state the obvious, and therefore there might be internal epitopes that exist in a protein that are not normally exposed by the native protein. Recall that we were dealing with the issue of how the immune system is able to create a wide diversity of antibodies, immunoglobulins would share in common a constant domain.
And the constant domain is formed by these invariant regions of the light and the heavy chains. We call this the heterotetramer in contrast to the variable domain up here which recognizes the antigen that initially provoked the production of this antibody molecule.
And, the immune system may at any one point in time be making hundreds of thousands, maybe even millions or tens of millions of distinct antibody species that differ one from the other by the antigen recognition site here at this part of the antibody molecule.
And recall as well that since these are proteins, the antigen-recognizing pocket is itself an oligopeptide, which combined in some complementary fashion to whatever antigen initially provoked the synthesis of this antibody molecule.
At the end of last time, we discussed the fact that antibodies are made by B cells, and the fact that in the disease of multiple myeloma, one ends up with a monoclonal disease, that is to say, one of a vastly heterogeneous population of B cells begins to proliferate uncontrollably.
And now, all of its descendants make a single antibody species.
And that single antibody species consists once again of a heavy and a light chain, whose identity is created by the antigen-recognizing pocket that it happens to carry. And this begins to suggest a notion of clonal expansion. By that, I mean the following.
Let's imagine a scenario where we start out with a naïve immune system where we have a whole series of different B cells.
And this series goes here from 1-15, but in fact this could go from one up to a million. And each of these antibody producing B cells, or each of these B cells, has in principle the ability to respond to a different antigen.
Let's just imagine that. And now, what we can imagine is that an antigen such as, for example, poliovirus comes into the body, and it is recognized by two different clones of these B cells. And that recognition, we can imagine, acts as a mytogenic signal, a growth stimulatory signal for these two particular clones and B cells. It almost acts as if it were a growth factor.
But of course, we're not talking about growth factors here.
We're talking about antigens, including antigens brought into the tissue by a foreign infectious agent. And therefore, the recognition of the antigen by these two B cells may thereafter provoke their clonal expansion. By that I mean to say that they will preferentially begin to proliferate whereas all the other B cells, which are not in any way responsive to that antigen will just sit there like bumps on a log. And, if there is, therefore, clonal expansion, now there will be a much larger number of cells in the immune system that are capable of producing the antibody that recognizes its provoking antigen.
Again, recall that this number may go up to a million.
This is just a great simplification. Now here, we use the word plasma cells. And in fact, if one wants to get the nomenclature very proper, the plasma cells are the products of B cells which mature into the antibody producing plasma cells.
They are very close on the same lineage of cells, and what that means in the end for us is if once there's an active immune response, there's been the preferential expansion of certain sub-populations of B cells and plasma cells, and other ones of them which don't recognize these antigens just sit there are underrepresented in the total population of cells.
Still, the fundamental question that we posed last time was the following. If it's the case, and it happens to be that each of these B cells makes an antibody having a distinct reactivity, a distinct ability to react to one or another antigen how do they make so many different kinds of antibody molecules. How do they know how to do that. We argued that it's implausible that the amino acid sequences of each antibody molecule are encoded in the germ line. Why? Well, if there are indeed a million or even 100 million distinct antibodies can be made by the immune system, clearly there can't be 100 million genes, each of which is dedicated to the production of a distinct antibody molecule. And the solution to this puzzle was first worked out by Susumu Tonegawa already more than 20 years ago who happens to be in our biology department. And what he discovered was the following, that the way that the antibody producing genes are organized is really quite extraordinary and unusual. Here's the heavy chain of the gene, and keep in mind that there's a heavy and a light chain gene.
The heavy and the light chain genes are in different chromosomes.
And therefore, the genes that are responsible for encoding these two proteins, the heavy and the light chain, they're on different chromosomes, and encode by different genes. OK, so what Tonegawa discovered was the following, that the variable region of an antibody molecule which is at its end terminus is encoded by small segments of DNA that are carried in one very large genetic locus, an immunoglobulin locus. And what happens in humans is the following. There are in humans rather than mice, this number should be 100 in humans, there are 100 distinct V segments that are encoded in this antibody locus. Each one is roughly the same size, and they're located in a large tandem array.
Similarly, there are in humans 30 of these desegments.
The numbers change here if one goes from mouse to human, but that's irrelevant. And, there are six of the J segments. And, much of the antigen-recognizing capacity, much of the variable region is encoded in the green and the blue region of the gene, and the encoding happens as follows.
There is a combinatorial fashion the random fusion of V, D, and J segments. There are specific, highly specialized enzyme that will choose one specific V segment, one specific D segment, and one specific J segment, ostensibly on a stochastic basis, a totally random basis, and fuse them together. And if that happens, and the intervening DNA sequences are discarded, then right away we can see that through the combinatorial mathematics that results from this, there is the possibility for having 100 times 30, which is 3, 00, times six, which is already 180, 00 distinct combinations encoding the reading frame of this particular antibody molecule. Here, I've just said V23, I guess, D7, J2. But again, let's imagine, which is approximately the case, that each of these combinations can be fused with roughly equal probability.
What happens subsequently, then, is that this variable region sequence, which has been formed by fusion, is then the transcript that comes from that is spliced to the constant region which is towards the C terminus. So here, when we talk about the V, D, and J segments, we're talking about the segments that are encoding this portion. Here's this portion of the antibody molecule.
Keep in mind, from here on down, we're talking about constant region segments which are not affected by these combinatorial fusions.
And, in the overhead I just showed you, I was just talking about how the heavy chain gene is rearranged. And what that results as a consequence is the following. This is just another version of what I just showed you before, where the V, D, and J segments become fused randomly to one another creating a V, D, J fusion thing, which then by splicing is fused to the constant region which here is called C sub-mu.
For reasons of why it's called sub-mu I'll mention in a moment.
And this is the actual messenger RNA which then goes into the cytoplasm. And what we can now imagine is the following, that when the immune system first begins, it randomly fuses whole series of V, D, and J segments together, and all kinds of combinations. The same happens in both the heavy chain and the light chain, although the light chain is slightly simpler. But that's irrelevant for us conceptually. And then, we end up having hundreds of millions of distinct V cells, each of which has a different combination of heavy and light chains because keep in mind the light chain genes are also being rearranged combinatorially.
They're also being fused. And therefore, we can have 180, 00 distinct heavy chains, and I forget the exact number for the number of light chains. It's a bit less, but we can multiply those two combinations by one another because keep in mind that the gene encoding the heavy chain here in its variable region, and the gene encoding the light chain in its variable region are organized on separate chromosomes. And, they're fused together. These segments are fused together randomly. So, the total number of antibody molecules we can make is 180, 00 distinct kinds of these.
And, I've just slipped up in knowing the total number of these that can be made, although we could figure it out shortly if it were really important. But once again, the number is in the many thousands.
And therefore, overall, the total number of distinct antibody molecules one can make on the basis of this is this.
Did I get the math right, 60 by 30, 18,000. It's 18, 00. So, there's 18,000 distinct of these that can be made, and the number of these, I think, is closer to 10,000.
I forget, but multiplied, let's call it 10,000 for a moment.
That's not the right number. There's 10,000 different ones of these, and 18, 00 of these that can be made.
Multiply them together, and you get the total number of combinations of antibody molecules it could make because it is the case that the antigen recognition site here is created cooperatively, collaboratively, by the heavy and light chain. You had a question?
So, the gene is rearranged, and when the rearrangement occurs, on V segment is fused to one D segment, is fused to one J segment.
And all of the extraneous segments that are between them have been deleted from the chromosome. So, this leads me to a second point I wanted to make, and that is that here we are dealing with a process of somatic mutation.
We talked about last time or the time before last the fact that somatic mutation is generally a process that can lead to cancer, i.e. the mutation of germ line genes that are in one way or another corrupted by random mutational processes. But here, I'm talking about a somatic mutational process, which is highly directed and highly organized, and focused on creating a series of rearranged genes that can then serve as the template for the production of an antibody molecule. So here, let's just look at this again. Here, I'll tell you, there are many V genes. There are many D genes, and there are many J genes. And, this is the embryonic DNA. After large segments of the immunoglobulin locus are deleted, and then the surviving V, D, and J segments become fused in the DNA directly to one another. And when that happens, the resulting B cell DNA can be transcribed, and via splicing create an mRNA where the region in between the J's chain and the constant region chain called, here C, is further deleted.
So, each messenger RNA has one V, one D, and one J segment together with a constant region segment. There are yet other processes that further diversify how many different variable regions can be encoded.
One of them is the following. It turns out that the process by which the V and D, and the D and J genes are fused together, those segments are fused together, is a bit sloppy.
And therefore, sometimes the fusion will occur creating a sequence of nucleotides that has a nonsense codon in. Sometimes it will create a coherent reading frame that creates yet another kind of amino acid sequence there. And therefore, this is not a highly ordered process in the sense that these micro-architecture of the points with the V and the D, and the D and the J are fused is a little bit chaotic. So that creates even further randomization of the nucleotide sequence. And then, finally, once this gene has been created, there is a process which is called hypermutation, where through mechanisms which are not well understood, the nucleotide sequence of this region is actually further changed.
It's mutated by certain enzymes. For example, there's an enzyme called cytidine deaminase, which takes off the amine group off of the cytidines in that region, and thereby effectively converts the C's in that coding region more into having a T coding capacity.
This happens somatically, and here once again I'm talking about a further dimension of diversification.
One of the dimensions diversification, first of all, the V and the J chains rearrange independently, randomly, and stochastically. Secondly, the joining of the segments is a bit sloppy. And thirdly, there is somatic hypermutation. There's an enzyme like this which is actually an actively mutagenic enzyme which fiddles around with some of the C's in this portion of the gene, thereby further diversifying the coding ability of the immunoglobulin gene that's encoded by the cell. And what this means is the following, that if we imagine this scheme here where each of these B cells recognize it's a different antigen, initially there may be 100 million distinct B cell clones that are created, each the consequence of a different random mutation. That happens early in the development of the immune system. Thereafter, there are certain selective processes there at play. One important selective process is the elimination of B cells that have failed to make nicely rearranged antibody molecules. What do I mean by that?
I told you how sloppy the V, D, J joining is. And therefore, many of these joinings could create reading frames that are incoherent with stop codons in the middle. And therefore, those cells which happen to have created antibody molecules which are highly mutant and clearly structurally defective are eliminated. So, one prerequisite for the survival of these B cells early in the development of the immune system is that they've learned how to make functional heavy and light chains.
If they don't, they're right away wiped out. They're eliminated.
Here's another very important prerequisite for survival, that these B cells don't make antibodies that react with antigens that are native to the body's own tissues. What do I mean by that?
Well, one of the wonders of the immune system is the following, that it can recognize foreign antigens that are brought into the body from the outside including, for example, the epitopes on the surface of polio virus particle. It can recognize many different viruses, surface antigens present on the surface of bacteria, and fungi, and all kinds of other infectious agents.
But importantly, the immune system also sees hundreds of millions of different epitopes of the proteins that are present endogenously, native proteins in our tissues. A priori, the native proteins, what's called our own self-proteins, are perfectly qualified to function as antigens.
And yet, the immune system rarely develops strong reactivity against them. Thus, the immune system has the ability to recognize self versus non-self. What do I mean by self versus non-self?
Self are the body's own native proteins, which are in principal conceivably antigenic. Non-cell are foreign invaders that bring strange epitopes into the cell.
And what happens is that B cells, which make antibodies, that recognize native proteins in our tissues are, if things are working well, rapidly eliminated early in the development of the immune system.
What happens if that elimination fails to occur properly?
If it fails to occur, one has the process of autoimmune disease.
And, there are multiple autoimmune diseases.
Type one diabetes, early onset diabetes happens when the immune system recognizes antigens that are present in the islet cells in the pancreas that make insulin. Rheumatoid arthritis, which inflicts a large portion of the elderly, happens when the immune system recognizes antigens that are present normally in the cartilage in our joints. Lupus happens when, it's an autoimmune called lupus which is often fatal, happens when the immune system recognizes proteins in many different tissues.
And once again, in all of these cases, there is a breakdown of the mechanisms governing immune tolerance. And what do I mean by tolerance? I mean the mechanisms whereby the immune system tolerates native antigens, but is conversely intolerant of foreign antigens.
The word intolerant is not used in immunology, but I'm just using it for the sake of explanation here. So, immune tolerance is a very important area of current immunological research.
We don't really understand why we don't have much more autoimmune disease than we do. And by the way, only listed two or three of the most common kinds of autoimmune diseases that happen with the mechanisms that normally guarantee immune tolerance failed.
And therefore, these B cells survive are ones that make functional antibodies and those whose antibodies do not recognize self-antigens. They are permitted to survive, and once again, we imagine that those that happened to make antibodies that recognize particular foreign antigens undergo or enjoy clonal expansion. During the development of the immune response, there is a further diversification of the antigen-recognizing domain of the protein by hypermutation.
And therefore, descendants of these initially developed cells, which have begun to expand because they make an antibody, may develop antibody molecules that are even more able to bind avidly to the antigen. It could be that initially, these antibody-producing cells make antibody molecules that bind nicely to the antigen but not really avidly. Avidly means really tightly. And, that's enough to get their clonal expansion going, but during the process of clonal expansion, this hypermutation creates further variants of these cells, further mutates their antibody producing genes so that some of the descendants of these cells will produce antibody molecules that bind even more tightly and specifically to the provoking antigen. And when that happens, the quality of the antibodies is improved progressively.
Now clearly, the somatic hypermutation once again, it's a random hypermutation. And those clones of cells in which the hypermutation created less effective antibodies will not have their proliferation stimulated. Those clones of cells whose antibodies make more and more tightly binding antibodies will preferentially have their proliferation stimulated.
And as a consequence, they will now be the ones that are favored to yield the plasma cells that produce large amounts of antibody molecules. So, we have now this very unusual and very interesting way by which B cells and plasma cells are able to create a wide diversity of antibody molecules. Now, one of the interesting things, actually, is the fact that this constant region contains one of a variety of distinct constant regions. Initially, when one produces the first immune response, the first immune response as we said before produces a constant region which is called CM, and I had the right overhead this morning. Ah, here it is.
Here's the way the genes are actually rearranged.
Here's the rearranged V, D, and J, and here's the splice to the constant region. And downstream are a whole series of constant region segments. What's the first one: CM.
I told you about that before. Thereafter, C sub delta, C sub gamma-3, gamma-1, gamma-2, gamma-8, and so forth.
And therefore, the initial event can create an ig-mu, an IgM antibody molecule. The mu region creates an IgM.
Later, as the immune response develops further, this mu segment may become deleted. And now, the splice may occur to a delta or a gamma change. And therefore, if the intervening constant region is changed, you might get what's called an IgG.
An IgG is an immunoglobulin which is produced when this antigen-recognizing region becomes spliced down to here or here by virtue of the deletion of these intervening constant region segments. What's the purpose of what's called class switching? Because these are different classes of antibodies, because they have different functions. Note importantly that when this class switching occurs, it has no effect on the antigen-recognizing site of the antibody molecule. Rather, it affects the constant region of the antibody molecule. Well, how does that work? The initially made antibody molecule, I told you, is IgM. And in fact, if you look at the structure of IgM, it looks like this. Here's, let's say, a B cell, and the IgM molecule is actually not secreted into the extra cellular space like a soluble antibody molecule. Here's what IgM looks like from very schematically IgM has our standard antigen-recognizing site, but IgM is embedded in the plasma membrane of the B cell exactly the way that a growth factor receptor is embedded in the plasma membrane of a B cell. But keep in mind that I just said that this IgM molecule has an antigen-recognizing domain up here, which is exactly the way we described it before. So, the antigen recognition is not affected by whether we have an IgM molecule here.
Only the location of the antibody molecule, and as I'll show shortly, the function of this antibody molecule is affected by this IgM.
Later on, descendants of this B cell will mature into those that secrete IgG molecules into the plasma.
So, here's a maturation that's going on. But look here.
Once again, the antigen-recognizing domains of this IgG are unaffected by this conversion. Here, the constant region is tethering the antibody molecule to the plasma membrane.
Here's the antigen-recognizing sites.
After this maturation occurs, the secreted IgG molecules, which go into the solution, have identical antigen-recognizing sites, but now they're soluble proteins. Well, you'll say, why does the immune system do that?
And, it goes back to an overhead I showed you just moments ago in which there was a puzzle implicitly created by the image on the overhead.
And here it is. Let's look at this for a moment.
What detail is now explained in this scheme? What detail is now explained? I told you that B cells can be stimulated by certain antigens. Let's imagine that each of these B cells made only secreted antibody, OK, instead of making cell surface antibody because the cell surface antibody, which is being made in this scheme, is actually the IgM molecules. Let's say this B cell over here is making an antigen. I'll just draw it as, there's the antigen, is making an antibody against this antigen, and it's secreting antibody against this. And I'd just argue that the B cell that makes good antibody is favored. It has its proliferation stimulated, right? That's what we talked about.
But if this B cell sends all of its antibody into the plasma, how is it going to know that it's making a good antibody?
It can't because all of the antigen recognition is happening by the secreted antibody. So, what happens with the IgM molecules. The first antibody molecule this produced is IgM.
And, it stays tethered to the plasma membrane of the B cell.
It's a transmembrane protein, and it functions much like a growth factor receptor. That is to say, when the antigen binds out here, there are signals that are radiated into the cell that induce the proliferation of this cell.
And now, these cells that produce the antigen-recognizing IgM molecules on their surface can now have their proliferation stimulated.
That solves the puzzle here which is created by this scheme.
If all these B cells just made secreted antibody like this, then there would be no way to encourage their proliferation because there would be no way of telling this B cell you're doing a good thing. Keep making more of these antibody molecules. Here, the antigen-recognizing capability is embedded in the plasma membrane. And when an antigen binds this, the IgM molecule's organized so that now growth stimulatory signals are sent into the cell which cause this cell to begin to proliferate, producing more IgM containing cells which are further stimulated to proliferate. And ultimately after this has gone on for a while, there is a class switching which deletes the IgM region of the gene chain region, and causes a conversion to IgG. Again, the conversion from here to here doesn't change the antigen-recognizing capability of these two antibodies. It just makes a cell surface transmembrane protein or a secreted protein. (pause) No, the VDJ choice happens in the nucleus. It's a fusion of different DNA segments, and when it's transcribed, that fused VDJ is now spliced to a constant region segment.
The choice of VDJ is a fusion of DNA segments. It happens in chromosomal DNA. It results in the deletion of all the segments that aren't used. So, all that's left in the immunoglobulin locus of a B cell is a DNA segment encoding V fused to a DNA segment encoding D, fused to a DNA segment encoding J.
So now, you have a new somatically mutated immunoglobulin gene, lest there be any residual confusion about that. So, you see the elegance of this class-switching thing.
Now there's yet other constant region segments that are used for other purposes. For example, when you have allergies, there's an activation of the igE antibodies.
When you have secreted antibody production in the colon, igA is used more for that. So, different ones of these constant regions are used for different immunological applications.
The IgM molecule, as I've said, is used here just as a way of telling the B cell that it's done well by making the right kind of antigen-recognizing site. The real business of creating antibodies is the soluble antibody production, the immunoglobulins, or the gammaglobulins because the vast majority of antibodies floating around the blood plasma are in fact IgGs. The IgMs are, as implied by this, actually just largely tethered to the surface of cells. So, this really is an extraordinary, elegant way of creating essentially hundreds of millions of different antigen-recognizing domains each created collaboratively between a heavy and a light chain. And once these antigen-recognizing domains are created through changes in DNA structure, then they can be used for different immunological applications, driving B cell proliferation, secreting soluble antibodies that are used to neutralize virus particles in the blood, or if they're hyperactive to create allergic reactions or to create immunity in certain regions of the gut and so forth.
So, this class switching doesn't change the antigen-recognizing capability. It just changes the utilization of how already-developed VDJ segments and their antigen-recognizing capability are exploited by cells of the immune system.
Now, one thing that's not really clear by all this is how all this develops at the cellular level because what I've talked about until now is called humeral immunity. Well, we talk about somebody having a good sense of humor. But the actual original meaning of the word humor in Latin was fluids that were fluxing through your body, and were responsible for your different mood states.
So, if you were depressed or there just was a national election, there would be black fluids, black humors coursing through your blood. And if you were in a good mood, there were other humors coursing through your blood.
And that leads through this etymology to the term of humeral immunity, which is to say the soluble immunity, i.e. the production of soluble antibody molecules.
But I will tell you that there's a second arm of the immune system which is equally important, and that's called cellular immunity.
And, to make a long story short, and we'll elaborate it on Monday briefly. Cellular immunity is largely created when you have a kind of immune cell that's called a cell rather than the B cells we've been talking about until now, which is able, and this cellular immunity is among other things, cytotoxic.
And, it can recognize a second cell over here. Here's a second cell.
This is its target cell because the second target cell is displaying on its surface certain antigens. So, antigens are being displayed on the surface of the target cell. I've indicated them here as just little, blue strokes. And the T cell is able to recognize these antigens on the surface of this cell here, and is able to kill this cell through a series of interesting mechanisms. This doesn't involve the intervention of soluble antibody molecules. Here, we're talking about one cell recognizing another, and it turns out to be very important for antiviral defenses. I've told you one way by which antiviral defenses are achieved. Soluble antibody molecules are secreted into the blood plasma like IgGs. They recognize an epitope on the surface of a virus particle. They glom onto that epitope on the surface of the virus particle, and they neutralize the virus particle. And, that's very important.
We started out in our discussion of poliovirus talking about that.
But this also turns out to be very important. Why is it important?
For the following reason: when this cell, let's say this cell is infected by poliovirus on the inside.
It turns out, interestingly enough, that the cell has a way which we'll discuss of processing poliovirus polypeptides, viral polypeptides, and displaying them on the cell surface. In other words, the cell can chew up some poliovirus proteins, put them to the outside, and tell the outside world, these are the kinds of the proteins that are being made right now inside of me.
You the immune system can't really look through the plasma membrane so I'm going to tell you this is what's going on inside of me.
There are poliovirus proteins being made as we speak.
On the outside of the cell, these are displayed even though poliovirus replication is recurring exclusively inside the cell.
A cytotoxic T cell here may recognize these antigens on the surface of the poliovirus infected cell, and proceed to kill the cell.
Why is that interesting or important? Because the cytotoxic cell will kill the virus-infected cell while the virus infection is still in full swing. And therefore, by killing the virus-infected cell, it will abort the entire bioreplication cycle because the virus won't have enough time to replicate or proliferate inside the infected cell before it happens. It's preemptively killed by the cytotoxic T cell. And in fact, this way of eliminating viral infections from our body is as important and often more important than the neutralization of soluble virus particles in our plasma.
And all that gets now to have us focus increasingly on the mechanisms by which antigens are recognized and processed so that the immune system can begin to respond to them. So, I want to get into that now, into the aspects in which we look at the cellular arms of the immune system. The most important thing initially in the immune response is that let's say poliovirus particle is recognized and digested by certain phagocytic cells of the immune system. What's a phagocytic cell: a cell that is able to gobble up other things. So, a well-known phagocytic cell is a macrophage. And there you can see the term phagocyte, macrophage. Phagos means to chew up or to swallow, so macrophage might see a particle like this surround that particle, and then internalize that particle and digest it, let's say a poliovirus particle. Macrophage could envelop a poliovirus particle and internalize it.
It could do the same thing with a bacteria. Here, we'll use as an abbreviation for macrophage this.
So, that's macrophage. There are yet other even more important phagocytic cells of the immune system that are called dendritic cells. Now, these cells gobble up whatever happens to be around them. They don't care.
They are promiscuous sewer, gutter trollers. Whatever happens to be around, they will gobble up whatever happens to be around.
They'll put it inside of them, and now they do something really interesting. The dendritic cells, the macrophages, the phagocytic cells, they don't digest the internalized particles down to amino acids. They digest the virus particle down to oligopeptides.
Usually if 10, 12, 14 amino acids long: very important.
So they don't make amino acids. They make oligopeptides. Why are they doing this digestion? Because these cells are exhibitionists and they want to show the rest of the world what they've just swallowed up. It's really important to them.
Go figure. So, what do they do?
They take these oligopeptides that they've just internalized and they put them on the cell surface via molecules which are called MHC class 2. MHC class 2 is the name of these molecules, and these are cell surface receptors. And, these MHT C class 2 molecules have included in them an oligopeptide that has just been produced by the proteolytic cleavage of an internalized infectious agent, let's say. And so, the macrophage has many of these MHC class 2 molecules on its surface, and it displays to the outside world what it has just captured in terms of these oligopeptides.
And these oligopeptides are, by the way, roughly the size of an epitope. Therefore, this macrophage is just so excited.
It's like a little kid who has just found something, so excited to show the world what it's just found and gobbled up and processed. And over here is a class of cells which are called T helper cells. And the T helper cells are interesting in their own right, right? The T helper cells have evolved through a mechanism that is similar to and parallel to the evolution of the B cells. As a consequence, there are many different kinds of T helper cells. Each one, so TH-1, TH-2, TH-3, each of which displays on its surface a T cell receptor.
What kind of T cell receptor does it display? A T cell receptor that has gone through exactly the same kind of hoops that the antibody genes have gone through. That is, each T cell expresses on its surface an antigen-recognizing receptor just like the IgM molecule except it's made by T cells and it's created by these stochastic processes of fusing DNA segments. So, each of these T cells, and again, there are millions of them, has a different T cell receptor with a different antigen-recognizing capability.
And here's what happens in the immune system.
Let's imagine, as I say every year, that we're in a Middle Eastern bazaar, and that Middle Eastern bazaar, here are all the shops on the sides on the sides of this long bazaar. It's sometimes called a shuk or a suk.
And here we're going to have a macrophage or a dendritic cell moving through the bazaar. Hanging out in front of the stores are a whole bunch of T helper cells.
Business is slow, so all these T helper cells are just hanging out in front, and as is not the custom in this region, let's pretend all the T helper cells are girls just for the heck of it. It doesn't make any difference. And each of these T helper cells is displaying its own T cell receptor which has arisen through stochastic mechanisms and which recognizes a different oligopeptide.
And here we have now the macrophage coming through like a vendor, a street vendor, saying, look girls, look at the epitope I just gobbled up and produced. Isn't it great? And, it's using its MHC class 2 molecules to present, it's like hands, presenting the epitopes. It's flogging them like a street vendor, and it walks down through this bazaar, and each of the T helper cells, they're congregating along the sides of the road, and most of them say look at this drip.
Look at the garbage he's selling us today. I am totally uninterested.
He was here last week with some other chump too.
Let's hope he disappears quickly. And meanwhile the macrophage or the dendritic cell is very anxiously trying to find someone who has even the slightest bit of affection or respect for him.
And this goes on for a long time.
And finally, the macrophage finds a T helper cell over here.
So, here's the T helper cell, and it turns out that by coincidence the T helper cell has on here surface a T cell receptor that recognizes the epitope that the macrophage is peddling.
So, here's the macrophage. Macrophage has on its surface this oligopeptide held by the MHC class 2 hands. So, here's the peptide.
And this T cell receptor which I'll abbreviate TCR recognizes, binds strongly to the epitope that's being flogged by the macrophage.
And this is, I will tell you honestly, love at first sight.
She says, oh, I can't believe it. You're selling an epitope that I just happen to love. This is very exciting.
And all the other T helper cells say, oh my God, what does she see in him? And she gets all excited because there's a direct complementarity. In fact, to tell the truth, the T cell receptor recognizes not only the epitope but also the surrounding amino acids in the fingers of the MHC class 2 molecule.
It's this constellation of things that's recognized by the T cell receptor and gets this T cell receptor really excited.
And what does she do? Well, we can't talk about everything because this is polite company. But most important for what she does is she begins to proliferate like mad. Well, you know, cells have a limited repertoire of behavioral routines.
Next time we're going to figure out how this leads to an immune response.
So, we're going to be on [intention? all weekend.
Free Downloads
Free Streaming
Video
- iTunes U (MP4 - 131MB)
- Internet Archive (MP4 - 131MB)
Audio
- iTunes U (MP3 - 12MB)
Caption
- English-US (SRT)